Immune tolerance induced by adoptive transfer of dendritic cells in an insulin-dependent diabetes mellitus murine model1
Introduction
Type I diabetes mellitus is a T cell-dependent autoimmune disease resulting in selective destruction of the β cells in pancreatic islets[1]. The loss of immune tolerance to β cell autoantigen leads to the generation of cytotoxic autoreactive T cells, which is the most important pathogenic aspect in the disease. Therefore, restoration of tolerance in pre-diabetic individuals may be a potential way to prevent disease development[2].
Antigen-presenting cells (APC) have been proven to be integral participants in the initiation and regulation of insulin-dependent diabetes mellitus (IDDM)[3]. Dendritic cells (DC) are a family of professional APC in the immune system and control immune responses to either augment or reduce autoimmunity by a variety of mechanisms, which is also related to their various subtypes or their state of maturation[4]. With respect to their immunomodulatory properties, it might be feasible to utilize DC for the development of intervention strategies to treat or prevent autoimmune diseases. Recent studies have suggested that in vitro cultured DC can serve as highly potent vaccines for various immunotherapies in experimental autoimmune model, such as experimental auto-immune encephalomyelitis and collagen-induced arthritis[5]. Clare-Salzler et al[6] demonstrated that the injection of DC isolated from draining lymph nodes of the pancreas (but not spleen DC) could protect non-obese diabetic (NOD) mice from disease development, but the detailed phenotype of injected cells was not specified in their study. To gain more insight into the effects of DC transfer in experimental autoimmune diabetes, we evaluated the effects of two distinct DC subpopulations from BM on the development of strep-tozotocin (STZ)-induced autoimmune diabetes. We show that treatment with immature DC (iDC) rather than mature DC (mDC) can confer protection to mice against IDDM induced by STZ, which is associated with enhanced differentiation of regulatory T cells.
Materials and methods
Animals and reagent Balb/c male mice aged between 6−8 weeks, weighing approximately 20 g (Experimental Animal Center, Tongji Medical College, Huazhong University of Science and Technology, Wuhan, China) were maintained in standard environmental conditions with free access to food and water. They were allowed to adapt to their environment for 1 week before the experiments were initiated.
STZ (Sigma, St Louis, MO, USA) was dissolved in sodium citrate buffer (pH 4.2) just before injection. The following reagents were used: RPMI-1640 (Gibco, Gaithersburg, MD, USA), ConA (Sigma, USA), Ficoll-Paque (Amersham, Uppsala, Sweden), MTT (Amresco, Solon, OH, USA); mouse granulocyte-macrophage colony-stimulating factor (GM-CSF) and interleukin-4 (IL-4) (R&D Systems, Minneapolis, MN, USA); fluorescein isothiocyanate (FITC)-conjugated anti-mouse CD4, Phycoerythrin (PE)-conjugated anti-mouse CD25 (eBioscience, San Diego, CA, USA); FITC-conjugated anti-mouse CD11c, FITC-conjugated anti-mouse CD86 and FITC-conjugated anti-mouse MHC II mAbs (BD Pharmingen, San Diego, CA, USA).
Generation of DC from BM The femurs and tibias from the Balb/c mice were collected under aseptic conditions and the marrow was flushed out. Mononuclear cells were prepared by grinding through a nylon mesh, depleted of erythrocytes with Ficoll lymphocyte isolation liquid, and suspended in RPMI-1640 [10% fetal calf serum (FCS)]; 3×107 cells were seeded into 6-cm dishes. Four hours later, the non-adherent cells were gently removed by swirling the dishes gently and aspirating the medium; and dishes were washed 4 times with phosphate buffered solution (PBS). Fresh RPMI-1640 medium containing 10% FCS, mouse GM-CSF (0.02 ng/L) with or without mouse IL-4 (0.02 ng/L) was added into the dishes. On every other day, the medium was removed and fresh medium with cytokines was added. The cells were harvested for study 7 d later.
Fluorescence-activated cell sorting (FACS) analysis of purified DC The two different DC cultured in GM-CSF or GM-CSF+IL-4 were collected in PBS (2×106cells/mL) and incubated with FITC-conjugated anti-mouse CD11c, FITC-conjugated anti-mouse CD86 or FITC-conjugated anti-mouse MHC II mAbs for 30 min at 4 °C, respectively. The cells were washed twice in PBS and analyzed with a flow cytometer (Becton Dickinson, San Jose, CA, USA) using CellQuest software (Becton Dickinson, San Jose, CA, USA).
Allogeneic mixed lymphocyte reaction (MLR) Allogeneic responder lymphocytes were prepared by passing mouse spleens through a nylon mesh, depleted of erythrocytes with Ficoll lymphocyte isolation liquid. Graded doses of DC and 2×105 T cells were cocultured as triplicate in 96-well plates in a total volume of 200 µL/well at DC/T cell ratios from 1:40 to 1:10. After 72 h, MTT (5 g/L) was added 4 h before the termination of the culture. Then 150 µL DMSO was added to each well and oscillated for 10 min. The absorbance was measured by an enzyme-linked immunosorbent assay plate reader (Multiskan MK3, Thermo Labsystems, Vantaa, Finland) at a wavelength of 570 nm.
Treatment of mice with DC The DC were washed 3 times in cold PBS before injection. GM-CSF DC or GM-CSF+IL-4 DC (3×105/mouse) were injected by ip route into the Balb/c mice, respectively. The untreated and STZ controls were administered with equal volumes of PBS. Subsequently, the STZ control and the mice treated with DC were injected ip with STZ 40 mg/kg for 5 consecutive days as described[7]. The mice were observed for the onset of diabetes with measurements of the glucose concentration in the blood obtained from a tail vein, which was performed weekly with a blood glucose detector (Yicheng Bioelectronic Technology Co, Ltd, Beijing, China). Consecutive readings of blood glucose levels >16.7 mmol/L were considered diagnostic of the onset of diabetes.
FACS analysis of CD4+CD25+ regulatory T cells The mice were sacrificed by cervical dislocation at d 28 after the last injection of STZ, and the spleens were removed aseptical-ly. The spleen cells, 2×106, were incubated with FITC-conjugated anti-mouse CD4 and PE-conjugated anti-mouse CD25 mAbs for 30 min at 4 °C. The cells were washed twice in PBS and analyzed with a flow cytometer.
Lymphocyte proliferation The spleen cells were treated with a red cell lysis buffer and total T cells were purified by non-adherence to nylon wool. CD4+ cells were positively selected magnetically with mAbs directly bound to MACS MicroBeads (Miltenyi Biotec, Bergisch Gladbach, Germany) according to the manufacturer’s protocols, and were counted as viable cells (Trypan blue stain negative). The proliferation of the CD4+ T lymphocytes was determined by MTT assay. Cells 2×106 treated with T lymphocyte mitogen ConA (5×10-3 g/L) were cultured in flat-bottomed 96-well plates in a total volume of 200 µL/well. After incubation for 3 d at 37 °C and 5% CO2, MTT (5 g/L) was added 4 h before the termination of culture. Then, 150 µL DMSO was added to each well and oscillated for 10 min. The absorbance was measured at a wavelength of 570 nm.
Histological examination of pancreata All of the mice were sacrificed at d 28 after the last injection of STZ. The pancreata were fixed in 10% neutral formalin (pH 7.0), processed for paraffin embedding, sectioned (5 µm), and stained with hematoxylin-eosin (H&E). The degree of insulitis was scored blindly by 2 independent observers using a semi-quantitative scale ranging from 0 to 4: 0, normal islet with no sign of T cell infiltration; 1, focal peri-islet T cell infiltration; 2, more extensive peri-islet infiltration, but with lymphocytes less than one-third of the islet area; 3, intra-islet T cell infiltration in one-third to one-half of the islet area; and 4, extensive intra-islet inflammation involv-ing more than half of the islet area. 8 to 12 islets from each animal were scored.
ELISA Serum samples of the mice were assayed for cyto-kine concentration. The levels of IL-2, interferon-γ (IFN-γ), IL-4, and IL-10 were determined using a mouse IL-2 ELISA kit (eBioscience, USA) and mouse IL-4, IL-10, IFN-γ ELISA kits (R&D systems, USA) according to the manufac-turer’s recommendations. Values are expressed as ng/L.
Statistical analysis Statistical analysis of mean values and difference were performed by Student’s t-test. A value of P<0.05 was considered statistically significant.
Results
Characteristics of DC To generate DC, the BM cells of Balb/c mice were cultured in the presence of GM-CSF, with or without IL-4. DC cultured in GM-CSF or GM-CSF+IL-4 were all 85%–95% positive for the DC marker (CD11c), 93.2% and 87.4%, respectively. The expression of CD86 (85.6%) and MHC II (87.5%) were significantly increased in GM-CSF+IL-4 DC. In contrast, DC cultured only with GM-CSF showed low expression of CD86 (19.4%) and MHC II (14.6%; Figure 1). Thus, based on these in vitro data, the 2 DC populations used in this study could be classified as iDC (GM-CSF DC) and mDC (GM-CSF+IL-4 DC) in agreement with the general concept of DC maturation.
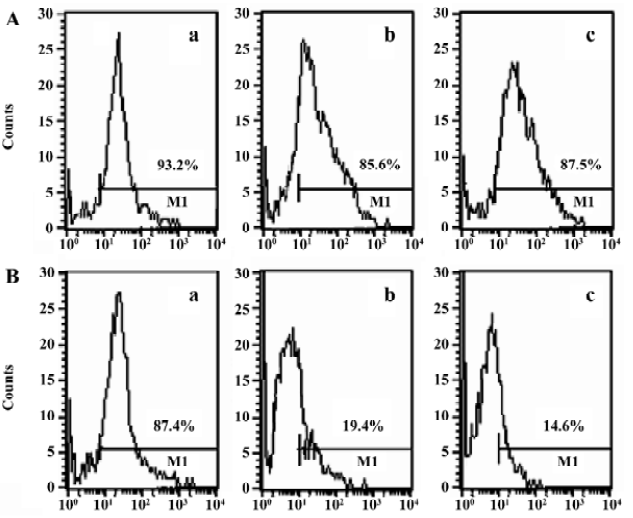
Stimulatory effect of DC in MLR The ability of the 2 DC populations on allogeneic responder lymphocyte was tested in MLR. The capacity of the DC subsets to stimulate allogeneic T cells was correlated with the levels of costimulatory molecules CD86 and MHC II of these 2 DC subpopulations. High T cell proliferation was induced by mDC from the ratio of 1:20 (stimulator:responder), whereas iDC elicited clearly lower T cell responses at whatever ratio (P<0.01, Figure 2).
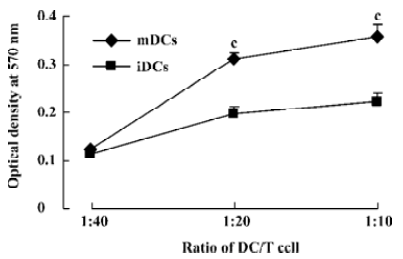
Protection of diabetes by iDC transfer The mice treated with multiple low doses of STZ became gradually hyper-glycemic. The disease occurred after injecting STZ with similar clinical appearances of human IDDM, such as increased hydroposia, food ingestion, urine and decreased bodyweight (data not shown). Recipients of iDC were significantly protected from diabetes (P<0.01), whereas mDC-treated mice did not avoid disease development; the level of blood glucose in these mice showed indistinctive differences with the STZ-treated mice (P>0.05; Figure 3). To test the effects of DC on the progression of insulitis, we examined the histology of pancreata of all mice. Pancreatic tissue sections from iDC-treated mice revealed a marked reduction in the degree and severity of insulitis compared to the STZ control, as judged by the ‘insulitis score’ (Figures 4, 5). We found that the beta cells of mDC-treated mice were destructed severely and there were large number of lymphocytes surrounding and intruding into the islets of Langerhans, with the similar condition of the diabetic mice. This is consistent with the above results of the blood glucose levels in Figure 3.
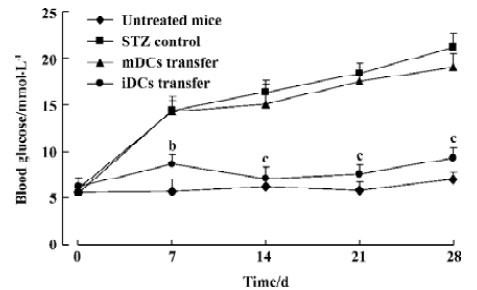
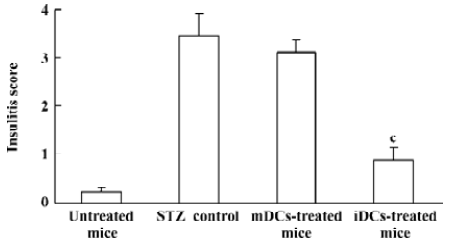
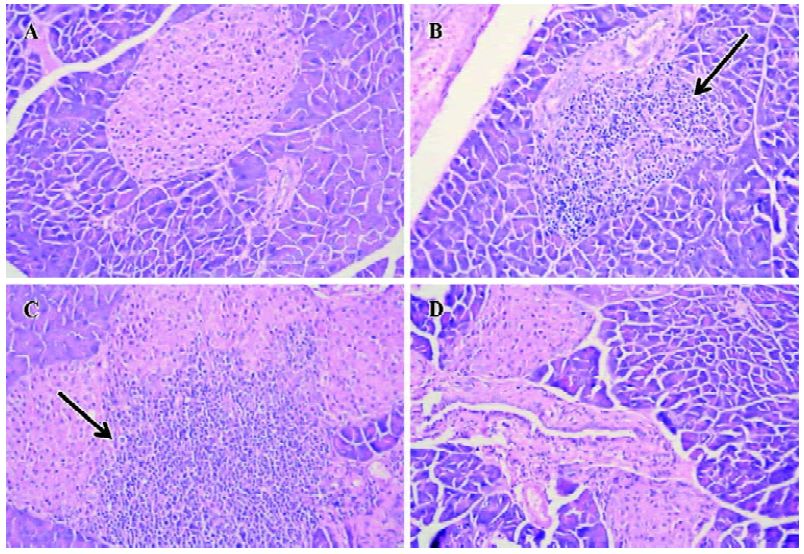
Enhanced proportion of CD4+CD25+ T cells by iDCs transfer To investigate the mechanism of the tolerogenic function of iDCs, we next analyzed whether this effect was correlated with enhanced accumulation or preservation the regulatory T cells. For FACS analysis of spleen lymphocytes we had determined the profile using fluorescently conjugated anti-CD4 and anti-CD25 Abs. We showed that there was a deficiency of CD4+CD25+ T cells in diabetic mice, while the normal proportion of these cells was 5% approximately. It appeared that the protective effect of iDC transfer might be partially due to the generation/activation of T cells within the CD4+CD25+ phenotype. In contrast, giving mDC did not improve the pathological state of these regulatory T cells (Figures 6, 7).
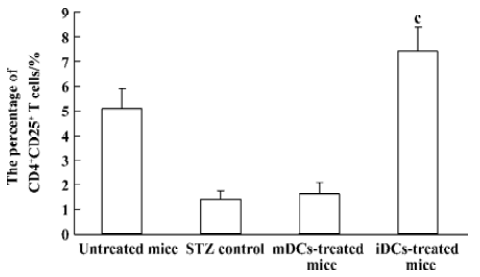
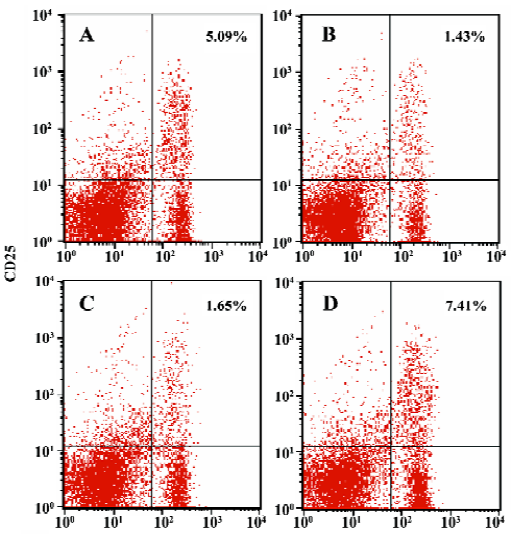
Decreased CD4+ T lymphocytes proliferation The effects of DC transfer on CD4+ T lymphocytes proliferation was examined by MTT assay. We observed increased lymphocytes proliferation in the STZ control, compared with the normal mice (P<0.05). The proliferative responses was significantly suppressed by iDC transfer (P<0.05), while there was no obvious change in mDC-treated mice (P>0.05, Figure 8).
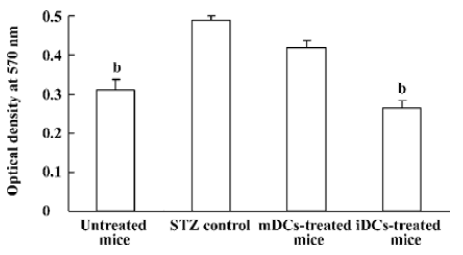
Cytokines shift by iDC To assess the effects of DC transfer on the production of Th1 and Th2 cytokines, the levels of IL-2, IFN-γ, IL-4, and IL-10 in the serum were measured by ELISA. Our results showed that transfer of iDC led to high amounts of IL-4 and IL-10 and low amounts of IL-2 and IFN-γ in the mice, whereas mDC did not, compared with the STZ control (Figure 9). These data showed that only iDC were able to induce a shift towards Th2 cytokine production after cells transfer into mice.
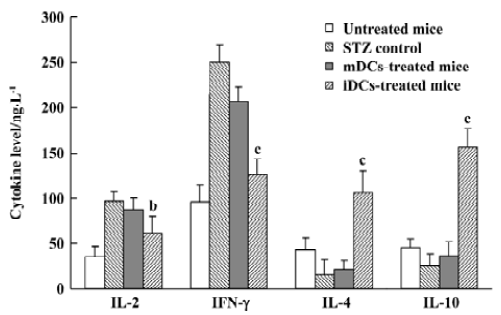
Discussion
IDDM is characterized by autoreactive lymphocytes infiltration in the pancreatic islet of Langerhans, followed by β cell destruction, leading to hyperglycemia. Multiple injections of low doses of STZ could develop an autoimmune diabetic model that exhibits many immunological and clinical features of IDDM in humans[8]. Injection of STZ in 5 equal low doses (40 mg·kg-1·d-1) induces a slow progressive hyper-glycemia, accompanied by lymphocytic infiltration of the pancreatic islets. This model provides a basis to understand the mechanisms involved in the onset and modulation of autoimmune pancreatic damage, which has been used extensively to study the immunological pathways that prevent insulitis and β cell destruction[9–11].
DC, as the professional APC, are uniquely able to either induce immune responses or to maintain the state of self-tolerance. Our work has been concerned with the 2 distinct DC populations derived from BM cells cultured in GM-CSF plus IL-4 or GM-CSF alone. Various in vitro studies have demonstrated that the culture of BM cells (myeloid progeni-tors) with GM-CSF results in the growth and differentiation of macrophages, neutrophils, and DC with immature phenotype. Furthermore, DC progenitors could be induced to develop into a large numbers of mDC by exposure to GM-CSF+IL-4[12,13]. Our data has described the differences in the phenotype and the T cell stimulatory capacity between the 2 DC populations. Activated mDC cultured in GM-CSF+IL-4 expressed high major histocompatibility complex (MHC) class II and costimulatory molecules. These cells were efficient stimulators of naïve allogenic T cells. In contrast, iDC expressing low MHC II and costimulatory molecules were poor T cell stimulators.
In the present study, we investigated the effects and mechanism of the 2 DC populations transfer to modulate the development and progression of diabetes in multiple low dose of STZ-induced diabetic mice. The first use of DC to prevent IDDM mice was documented by Clare-Salzler et al[6] who demonstrated that transfer of the pancreatic lymph node (PLN) DC derived from NOD mice into pre-diabetic NOD mice conferred significant protection from IDDM, while DC isolated from other lymph organs were not. According to the authors, the protections may occur because PLN DC carry a relevant islet peptide that is presented to lymphocytes and promotes the activation of regulatory elements. However, the phenotypes of these DC were not described, probably because of the small number of cells collected from PLN. Machen J et al[14] reported that a protective effect of DC against the development of diabetes was induced following injection of ex vivo antisense oligonucleotides-pulsed BM-derived DC, which suggests that the protective effect could be due to the state of DC rather than the tissue origin of DC. Growing evidence shows that iDC were able to induce T cell energy or differentiation of regulatory T cells, suggesting that the tolerance can be mediated by an immature stage of DCs[15–17]. These iDC are usually called ‘tolerogenic DC’[18]. Thus, such cells with tolerogenic property might prove to be useful tools for treating autoimmune diabetes.
Indeed, significant protective effect against IDDM was observed in the mice given a single injection of 3×105 iDC, but not mDC. The lower blood glucose levels were paralleled by the prevention of insulitis, which was demonstrated by examining the histology of the pancreata of the mice. We found a marked reduction of insulitis in the H&E sections (Figures 4, 5). These data indicate that injection of iDC was able to prevent the infiltration of immune cells that otherwise impair β cell function and promote β cell destruction. When DC were transferred to the mice who had already received STZ-injections, the anti-diabetogenic effect was not remarkable (data not shown), suggesting that the transferred DC can exert their anti-diabetogenic effect before stimulation of the self-reactive T cells by multiple low doses of STZ in the pancreatic islets.
Furthermore, we found an increased number of CD4+CD25+ T regulatory cells in the spleens of iDC-treated mice compared with diabetic mice, which might be responsible for protection against diabetes. We hypothesized that iDC circulated and took up dying cells derived from normal cell turnover. Upon phagocytosis in the absence of inflammation, DC remained immature, but were still able to migrate to regional lymph nodes. Then naive T cells in the lymph nodes may encounter antigen on these iDC, receive a suboptimal signal, and differentiate into T regulatory cells rather than effector T cells. As multiple low doses of STZ can induce inflammatory reactions in mice[9], iDC may be activated to express maturation markers in the local microenvironment and become unable to expand T regulatory cells. Therefore, administration of DC after STZ-injections could not be effective against diabetes.
CD4+CD25+ T regulatory cells appear to play an important role in controlling the progression of type 1 diabetes because a low level of CD4+CD25+ T cells correlates with exacerbation and acceleration of the disease[19]. In the NOD mice and IDDM patients, defects in the differentiation of the regulatory T cells that maintain peripheral tolerance to autoantigens probably contribute to disease development[20–21]. Our previous study also demonstrated that there are some abnormalities in the proportion of CD4+CD25+ T cell subsets in multiple low doses of STZ-induced diabetic mice[22]. iDC have been shown to induce profound accumulation of the regulatory CD4+CD25+ T cell subset. The shift of cytokine production towards Th2 profiles by iDC also appears to be associated with the increased CD4+CD25+ T cell population. CD4+CD25+ T regulatory cells could enhance regulatory Th2 response, inhibiting the Th1-mediated β cell destruction[23]. Protective Th2 cells produced high levels of IL-4 and IL-10, which may even contribute to the protection. Thus, iDC administration restored the balance between pathogenic Th1 and regulatory Th2 cells in vivo by the CD4+CD25+ T regulatory cells. In addition, CD4+CD25+ T cells inhibit the response of conventional CD4+ T cells in a contact-dependent manner and simultaneously confer suppressive properties to such cells, resulting in suppression of the proliferation of autoreactive T cells[24]. Thus, the generation or survival of regulatory cell activity in the splenocyte fraction may be a primary role of the protective effects of iDC in recipients.
In summary, data from the present study indicate that adoptive transfer iDC can confer protection to mice from STZ-induce diabetes. However, the DC subset, characterized by mature phenotype, failed to modulate the disease. The immunoprotection of iDC may be related to establishing immunotolerance by promoting the differentiation of CD4+CD25+ T cells and enhancing Th2 cytokine expression in vivo. Our results may have important implications for the development of therapeutical protocols based on DC transfer for the prevention of autoimmune diabetes.
References
- Yu LY, Lin B, Zhang ZL, Guo LH. Direct transfer of A20 gene into pancreas protected mice from streptozotocin-induced diabetes. Acta Pharmacol Sin 2004;25:721-6.
- Raz I, Eldor R, Naparstek Y. Immune modulation for prevention of type 1 diabetes mellitus. Trends Biotechnol 2005;23:128-34.
- Banchereau J, Steinman RM. Dendritic cells and the control of immunity. Nature 1998;392:245-52.
- Wakkach A, Fournier N, Brun V, Breittmayer JP, Cottrez F, Groux H. Characterization of dendritic cells that induce tolerance and T regulatory 1 cell differentiation in vivo. Immunity 2003;18:605-17.
- Xiao BG, Huang YM, Yang JS, Xu LY, Link H. Bone marrow-derived dendritic cells from experimental allergic encephalomyelitis induce immune tolerance to EAE in Lewis rats. Clin Exp Immunol 2001;125:300-9.
- Clare-Salzler MJ, Brooks J, Chai A, Van Herle K, Anderson C. Prevention of diabetes in nonobese diabetic mice by dendritic cell transfer. J Clin Invest 1992;90:741-8.
- Holstad M, Sandler S. A transcriptional inhibitor of TNF-γ prevents diabetes induced by multiple low-dose streptozotocin injections in mice. J Autoimmun 2001;16:441-7.
- Pechhold K, Patterson NB, Blum C, Fleischacker CL, Boehm BO, Harlan DM. Low dose streptozotocin-induced diabetes in rat insulin promoter-mCD80-transgenic mice is T cell autoantigen-specific and CD28 dependent. J Immunol 2001;166:253-9.
- Yang Z, Chen M, Fialkow LB, Ellet JD, Wu R, Nadler JL. The novel anti-inflammatory compound, lisofylline, prevents diabetes in multiple low-dose streptozotocin-treated mice. Pancreas 2003;26:e99-104.
- Mensah-Brown EP, Stosic Grujicic S, Maksimovic D, Jasima A, Shahin A, Lukic ML. Downregulation of apoptosis in the target tissue prevents low-dose streptozotocin-induced autoimmune diabetes. Mol Immunol 2002;38:941-6.
- Liu B, Zhang ZL, Yu LY, Guo LH. CMV-hFasL transgenic mice are sensitive to low doses of streptozotocin-induced type I diabetes mellitus. Acta Pharmacol Sin 2003;24:1199-204.
- Morel PA, Vasquez AC, Feili-Hariri M. Immunobiology of DC in NOD mice. J Leukoc Biol 1999;66:276-80.
- Hirano A, Fraser MO, Jordan ML, Takayama T, Lu L, Thomson AW. Graft hyporeactivity induced by donor-derived dendritic cell progenitors. Transplant Proc 2000;32:260-4.
- Machen J, Harnaha J, Lakomy R, Styche A, Trucco M, Giannoukakis N. Antisense oligonucleotides down-regulating costimulation confer diabetes-preventive properties to nonobese diabetic mouse dendritic cells. J Immunol 2004;173:4331-41.
- Gregori S, Casorati M, Amuchastegui S, Smiroldo S, Davalli AM, Adorini L. Regulatory T cells induced by 1 alpha, 25-dihydroxyvi-tamin D3 and mycophenolate mofetil treatment mediate transplantation tolerance. J Immunol 2001;167:1945-53.
- Lechler R, Ng WF, Steinman RM. Dendritic cells in transplantation−friend or foe? Immunity 2001;14:357-68.
- Canning MO, Grotenhuis K, de Wit H, Ruwhof C, Drexhage HA. 1-alpha, 25-Dihydroxyvitamin D3 (1, 25(OH)(2)D(3)) hampers the maturation of fully active immature dendritic cells from monocytes. Eur J Endocrinol 2001;145:351-7.
- Adorini L, Penna G, Giarratana N, Uskokovic M. Tolerogenic dendritic cells induced by vitamin D receptor ligands enhance regulatory T cells inhibiting allograft rejection and autoimmune diseases. J Cell Biochem 2003;88:227-33.
- Shevach EM. Regulatory T cells in autoimmunity. Annu Rev Immunol 2000;18:423-49.
- Serreze DV, Leiter EH. Defective activation of T suppressor cell function in nonobese diabetic mice. Potential relation to cytokine deficiencies. J Immunol 1988;140:3801-7.
- Salomon B, Lenschow DJ, Rhee L, Ashourian N, Singh B, Sharpe A, et al. B7/CD28 costimulation is essential for the homeostasis of the CD4+CD25+ immunoregulatory T cells that control autoimmune diabetes. Immunity 2000;12:431-40.
- Xiang M, Zhang CL, Zou XL, Cai XH, Peng JB. Roles of dendritic cells and regulatory cells in autoantigen-induced murine immune tolerance model. World Chin J Digestol 2006;14:687-92.
- Hoyne GF, Dallman MJ, Lamb JR. T-cell regulation of peripheral tolerance and immunity: the potential role for Notch signalling. Immunology 2000;100:281-8.
- Stassen M, Schmitt E, Jonuleit H. Human CD4+ CD25+ regulatory T cells and infectious tolerance. Transplantation 2004;77:s23-25.