Endogenous histamine inhibits the development of morphine-induced conditioned place preference1
Introduction
Morphine remains one of the most valuable drugs for the treatment of severe pain despite a large number of adverse side effects. The major drawback of their continuous use is the development of dependence, which is ascribed to their rewarding effects. These effects have been demonstrated in animals by the conditioned place preference (CPP) para-digm[1,2], which is based on the principle that, when a primary reinforcer is paired with a contextual stimulus, the contextual stimulus itself acquires secondary reinforcing properties. These secondary reinforcing properties can elicit place preference, which results in a significant increase in the time spent in the drug-paired place. The sensitivity of the CPP paradigm appears to be as great as or greater than those of other behavioral tests used to measure the rewarding effects of drugs[3]. However, in most studies to date, CPP is only tested after several conditionings of drugs paired with contextual stimulus. The process by which morphine-induced CPP develops is less well understood.
Histamine plays important roles as neurotransmitter and neuromodulator in the mammalian brain. Through its H1, H2 and H3 receptors, histamine participates in many physiological and behavioral functions including the sleep-wake cycle, emotion, locomotor activity, stress-related behavior, learning and memory[4,5]. The tuberomammillary nucleus (TM) is the only source of histaminergic neurons, which project to various parts of the brain including the nucleus accumbens (NAc) and the ventral tegmental area (VTA), regions known to be involved in reward mechanisms[6,7].
A role for brain histamine in regulating reward mechanisms has been recently documented in several studies[8]. For example, histamine injected into the lateral ventricle immediately raises the reinforcement threshold, which can be prevented by the application of histaminergic antagonists[9]. Histidine, a histamine precursor, attenuates the threshold-lowering effects of pentazocine[10]. H1 receptor antagonists act as rewards in combination with opioids[11] and even when given alone[12]. Therefore, it is likely that histaminergic pathways exert inhibitory control over reinforcement. However, little is known about the effect of endogenous histamine on the development of the reinforcement process in CPP induced by morphine.
Therefore, the present study was designed to investigate the involvement of endogenous histamine in the development of morphine-induced reward-seeking behavior, by using histidine (to increase endogenous histamine content) and TM lesions (to damage histaminergic neurons and decrease endogenous histamine content).
Materials and methods
Animals All experiments were carried out in accordance with the National Institutes of Health Guide for the Care and Use of Laboratory Animals. Male Sprague-Dawley rats (220–280 g, Grade II, Certificate N
Conditioned place preference Place conditioning studies were conducted in a apparatus consisting of a shuttle box which was divided into two equal-sized compartments (30 cm×30 cm×30 cm). The two compartments had different tactile and visual cues. One compartment was white with a smooth floor and the other was black with a wire mesh floor. The apparatus was used in dim illumination (40 lux) with masking white noise. A camera mounted above the apparatus allowed observation of each animal’s behavior on a video monitor.
Baseline preferences were assessed by placing the animals in the apparatus and allowing free access to both compartments for 15 min before drug administration. The time spent in each compartment was calculated for each animal and then averaged across the entire animal group. The animals showed preference for the black compartment, so we paired the morphine injections with the white compartment. Conditioning and testing then took place over the next 7 d (Figure 1). The rats were injected ip with saline on d 1, 3 and 5 and the drug at the designated doses on d 2, 4 and 6 of the experiment. The control rats always received saline. After morphine or saline administration, the rats were confined to the compartment designated as saline-paired or morphine-paired for 60 min. Morphine was always paired with the white compartment. Testing occurred on d 3, 5 and 7 following each conditioning session (1 saline-conditioning day and 1 morphine-conditioning day) and before receiving that day’s saline injection. The rats were handled on the test day in the same manner as the pretest.
Immunohistochemistry experiment Under deep anesthesia with chloral hydrate (360 mg/kg, ip), the rats that received an injection of 10 mg/kg morphine were perfused transcardially with 100 mL saline followed by 200 mL 4% paraformaldehyde in 0.1 mol/L phosphate buffer (PB; pH 7.4) 30 min after the end of the final preference test. The brains were removed and fixed in 4% paraformaldehyde for 2–4 d. Before immunofluorescence staining, each brain was immersed in 20%–30% sucrose in 0.1 mol/L PB(pH 7.4) until it sank. Then, on a freezing microtome (Leica, CM1900, Heidelberger, Germany), the coronal sections (30 μm) were cut consecutively through the TM nuclei, collected in 0.01 mol/L phosphate buffered saline (PBS; pH 7.4), washed 3 times for 5–10 min each, and pre-incubated with a blocking solution of PBS containing 1% bovine serum albumin and 4% normal goat serum (Vector Labs, Burlingame, CA, USA) for 1 h at room temperature. Sections were then incubated for 24 h at 4 ºC with mouse anti-histidine decarboxylase (HDC) monoclonal antibody (Sigma, St Louis, MO, USA) diluted 1:1500 in PBS with 2% normal goat serum and 0.25% Triton-X 100. After washing 3 times, the sections were incubated for 2 h at room temperature in the dark in Alexa 488-conjugated secondary antibody (goat anti-mouse antibody; Molecular Probes, Eugene, OR, USA) diluted 1:500 in PBS. The sections were then washed 3 times, mounted and examined under a fluorescence microscope (Olympus, BX51, Tokyo, Japan).
Neuron counting and measurements of perimeters were performed in sections from the saline- and morphine-treated brains. The outline of each HDC-positive neuron, together with the outline of the TM region, was drawn from all sections per brain. The number of HDC-positive neurons per section in each TM region was determined. The numbers of neurons derived from sections through the same brain were summed and divided by the number of sections. The perimeter of a HDC-positive neuron was measured using Meta-Morph image analysis software (Universal Imaging Corpora-tion, Downingtown, PA, USA). The length of the line outlining the neuron was used to assess the perimeter of HDC-positive neurons.
Measurements of histamine, glutamate, γ-aminobutyric acid (GABA), dopamine (DA) and 3,4-dihydroxyphenyl-acetic acid (DOPAC)
Sample preparation The rats were decapitated 30 min after the final CPP test. The brains were quickly removed, placed on an ice-cold stainless steel plate, and the VTA and NAc were dissected according to the atlas of Paxinos and Watson[13]. The tissues were stored at -80 ºC until assayed, when the samples were homogenized in 3% perchloric acid containing 5 mmol/L disodium ethylenediaminetetraacetic acid (EDTA) and 5-hydro-Nω-methyltryptamine in a Polytron homogenizer (Kinematica, Lucerne, Switzerland) at the maximum setting for 20 s in an ice bath. The homogenate was centrifuged at 15 000×g for 20 min at 4 ºC, then the supernatant was removed and filtered through a 0.22 µm polyvinyli-dene difluoride membrane.
Histamine, glutamate and GABA chromatography Histamine levels were analyzed by HPLC combined with electrochemical detection according to our previously published method[14]. In brief, the system consisted of a model 582 pump, a model 540 autosampler and a 4-channel CoulArray electrochemical detector. The HPLC was controlled and the data acquired and analyzed using CoulArray® software. All equipment was from ESA Biosciences Inc (Chelmsford, MA, USA). After reacting with the derivative o-phthalaldehyde, analytes were separated on a 3 µm, 3 mm×50 mm Capcell Pak MG C18 column (Shiseido, Tokyo, Japan). A two-component gradient elution system was used, with component A of the mobile phase being 100 mmol/L Na2HPO4, 13% acetoni-trile, and 22% methanol (pH 6.8) and component B being similar, but with 5.6% acetonitrile and 9.4% methanol. The gradient elution profile was: 0–3.5 min, isocratic 100% B; 3.5–20 min, linear ramp to 0% B; 20–22 min, isocratic 0% B; 22–23 min, linear ramp to 100% B; and 23–30 min, isocratic 100% B (flow rate 0.75 mL/min). The first cell was set at +250 mV and the second at +350 mV. All standards were obtained from Sigma (USA). Under these conditions, the retention times of glutamate, GABA and histamine were 5.58, 15.16, and 18.36 min, respectively. The detection limits (signal/noise ≥3) were 0.1 µg for glutamate, 5 ng for GABA, and 1 ng for histamine. Values were expressed as nmol/g wet tissue.
DA and DOPAC chromatography DA and DOPAC levels were determined with the above HPLC system, using methods previously described[15]. The mobile phase consisted of 71.5 mmol/L citric acid, 78.3 mmol/L citric acid trisodium salt, 832 µmol/L 1-octanesulfonic acid sodium salt, 107 µmol/L EDTA, pH 5.6 with 6% methanol. DA was separated on a reverse-phase column (HR-80, 4.6 mm×80 mm, 3 µm, ESA, USA) at 30 ºC and quantified by a 4-channel CoulArray electrochemical detector. The cell potentials were E1, -5 mV, and E2, +220 mV (flow rate 0.60 mL/min). DA and DOPAC levels were expressed as nmol/g wet tissue.
Surgical procedures Under chloral hydrate anesthesia (360 mg/kg, ip), the rats were fixed in a stereotaxic frame (Narishige, SR-5, Tokyo, Japan), and bipolar stainless steel electrodes (tip diameter 0.2 mm) were implanted bilaterally into the TM (AP -4.2 mm, L ±1.5 mm, and DV -9.6 mm). An electrolytic lesion was made by applying 1 mA DC for 10 s. In the case of a sham lesion, the electrodes were implanted into the same locations and connected to the lesion device, but no current was passed. At the end of the experiments, the animals were sacrificed and the locations of the electrodes were verified histologically. Only animals with electrodes located in the TM were included in the subsequent analysis.
Drugs Morphine hydrochloride (First Pharmaceutical Factory of Shenyang, Shenyang, China) and histidine hydrochloride (Sigma, USA) were dissolved in saline. Histamine, glutamate, GABA, DA and DOPAC were purchased from Sigma (USA).
Statistical analysis Data were analyzed with GraphPad Prism 4 (GraphPad Software Inc, San Diego CA, USA) and expressed as the mean±SEM. Place preference data were evaluated by two-way ANOVA followed by the Bonferroni test as a post test. Neurochemical data were evaluated by one factor ANOVA, followed by the Bonferroni test as a post test. Differences in histamine levels, DOPAC/DA ratios and the numbers and perimeters of HDC-positive histaminergic neurons between the saline- and morphine-treated groups were analyzed by Student’s t-test. Statistical significance was set at P<0.05.
Results
Effect of morphine on the development of CPP In the baseline preference testing, animals showed a preference for the black compartment, where they spent 831.59±21.31 s. CPP was affected by the morphine dose (F4,154=10.81; P<0.01) and the number of conditioning sessions (F2,154=3.69; P<0.05), but there was no interaction between these variables (Figure 2). An analysis of each conditioning session revealed that the rats treated with 2 mg/kg morphine showed significant CPP relative to the saline-treated group at its third conditioning session; 5 mg/kg morphine had similar effects at the second and third conditioning sessions, and a dose of 10 mg/kg showed significant CPP after the first treatment.
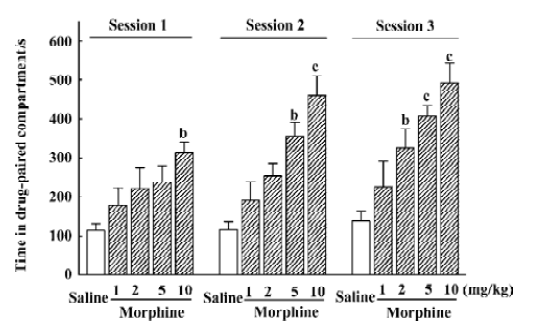
Effect of morphine on histamine levels in the VTA and NAc, and morphology of histaminergic neurons in the TM After 10 mg/kg morphine administrations, histamine levels in the VTA and NAc significantly decreased (VTA: P<0.01; NAc: P<0.01; Figure 3C). Histamine levels decreased to 47.4% in the saline-treated group in the VTA and to 43.0% in the saline-treated group in the NAc. In addition, green fluorescent protein-tagged HDC-positive (histaminergic) neurons exhibited considerable variability in shape compared with those from the saline-treated rats. Furthermore, HDC-positive neurons were smaller and sparser in the morphine-treated rats (Figure 3A). An analysis of the HDC-positive neurons from the saline- and morphine-treated rats revealed that morphine reduced both their mean number and size (Figure 3B).
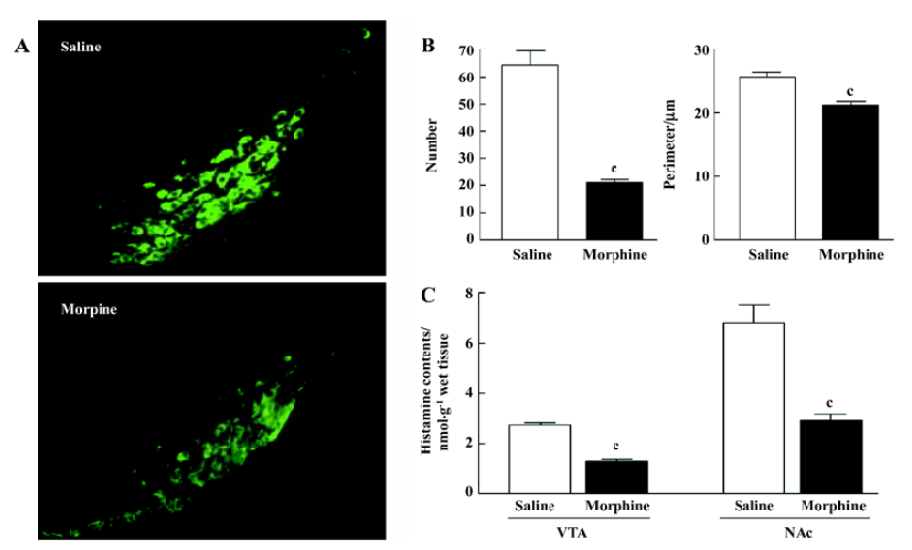
Effect of morphine on and DOPAC/DA ratios in the VTA and NAc The DOPAC/DA ratio is an index of the activity of dopaminergic neurons[16,17]. We found that morphine administration significantly decreased DA levels and elevated DOPAC/DA ratios in the VTA and the NAc (P<0.05; Table 1).
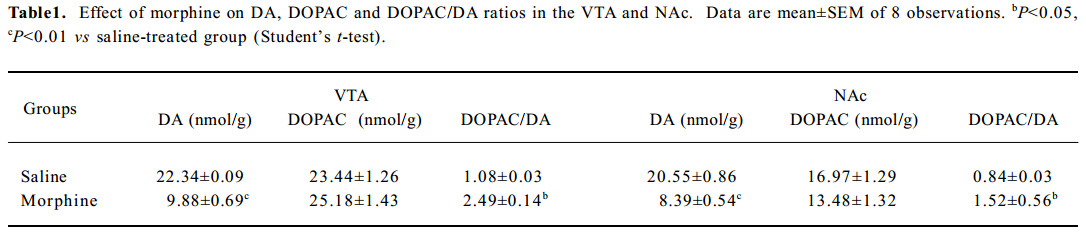
Full table
Effect of histidine on the development of morphine-induced CPP Morphine-induced CPP depended on the histamine dose (F3,125=12.29; P<0.01) and number of conditioning sessions (F2,125=15.19; P<0.01), but there was no interaction between these factors (Figure 4). An analysis of each conditioning session revealed that histidine significantly inhibited the development of morphine-induced CPP at doses of 100 or 200 mg/kg (P<0.05) during the first and second conditioning sessions, while during the third conditioning session, only 200 mg/kg histidine was effective (P<0.05). Histidine at the high dosage of 200 mg/kg did not induce conditioned place aversion when given alone (data not shown).
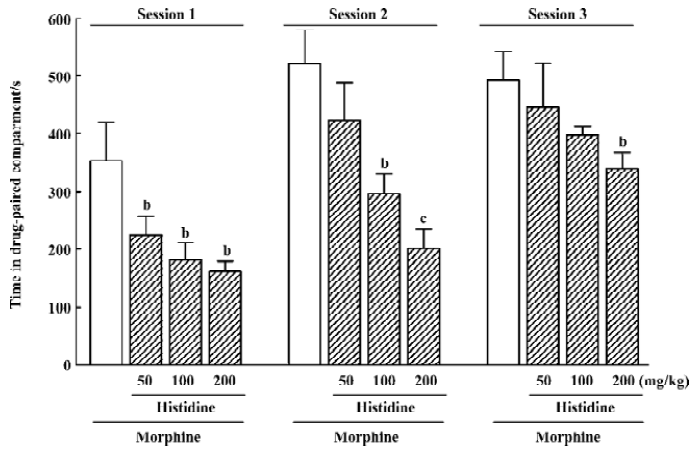
Effect of TM lesions on histamine levels in the VTA and NAc TM lesions affected histamine levels in the VTA (F3,32=12.91; P<0.01) and the NAc (F3,32=18.30; P<0.01; Figure 5). The histamine levels in the VTA and the NAc of the TM lesioned group were markedly decreased relative to the sham group; however, while the administration of morphine (1 mg/kg) to the TM lesioned group had no further effect on histamine levels in the VTA and NAc, histidine markedly increased histamine levels in these nuclei in the TM lesioned group exposed to morphine.
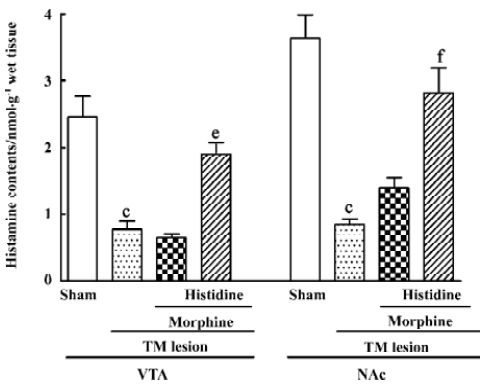
Effect of TM lesions on the development of morphine-induced CPP A two-way ANOVA showed significant effects of the experimental group (F5, 185=12.76; P<0.01), but there was no effect on the number of conditioning sessions or interaction between these variables (Figure 6). Morphine (1mg/kg), TM lesions alone, or sham with morphine had no appreciable effect on the development of CPP, but TM lesions with morphine induced significant CPP relative to sham combined with morphine from the first to third conditioning sessions, while histidine inhibited the CPP induced by TM lesions combined with morphine at the second and third conditioning sessions.
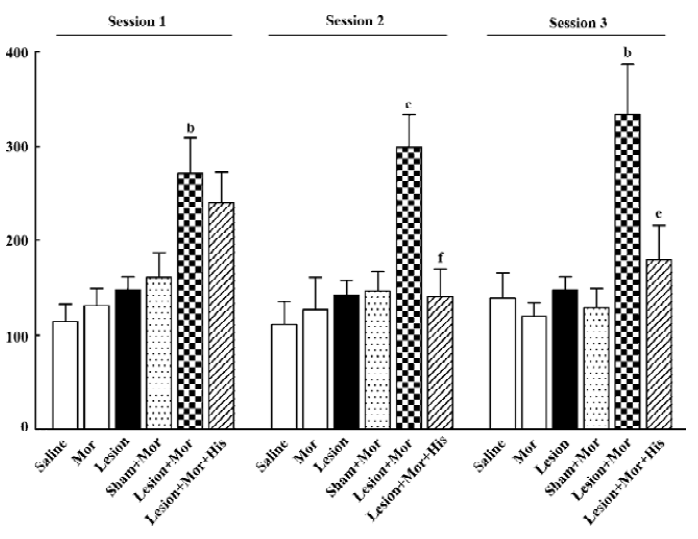
Effect of TM lesions on glutamate and GABA levels and DOPAC/DA ratios in VTA and NAc Treatment with morphine, histidine or TM lesions did not change the glutamate or GABA levels in the VTA or the NAc (Table 2). The TM lesion significantly decreased DA and DOPAC levels in the VTA, and decreased DA levels in the NAc (Table 3). After TM lesions, an analysis revealed groups treated with morphine or histidine changed the DOPAC/DA ratios in the VTA (F3, 32=11.76; P<0.01) and NAc (F3, 32=27.51; P<0.01; Table 3). In the VTA, TM lesions changed the DA/DOPAC ratio; lesions combined with morphine increased the ratio, but histidine did not affect this increase. However, in the NAc, the DA/DOPAC ratio significantly increased after TM lesions and lesions combined with morphine markedly increased the ratio, but histidine significantly reversed this increase.
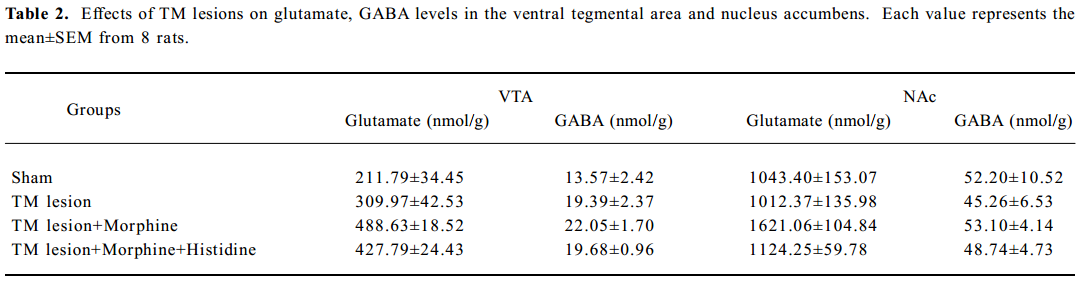
Full table
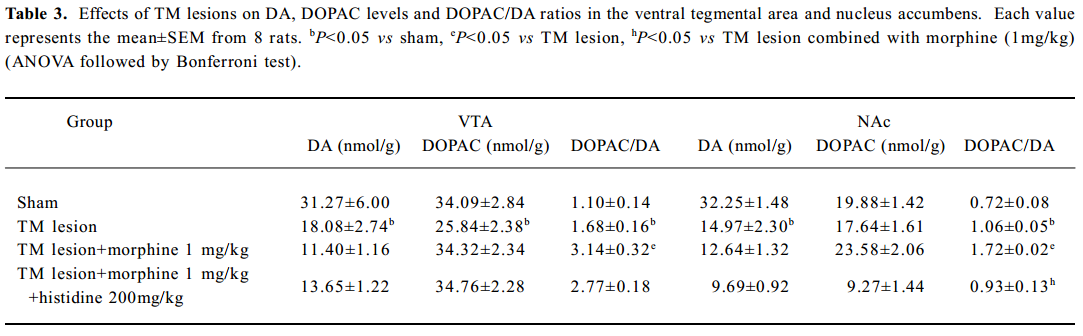
Full table
Discussion
Morphine is abused for its rewarding and/or euphoric effects, and in animal models, the rewarding effects are demonstrated by the ability to induce CPP[18]. In the present study, we found that morphine dose-dependently induced the development of CPP, and 3 administrations of morphine at 10 mg/kg induced significant CPP, as previously shown by others[19]. We therefore continued to use this protocol in the present experiments.
The VTA and the NAc are the most likely sites of action for morphine-induced CPP[20,21], so we analyzed the histamine levels in these nuclei after morphine administration. Histamine levels significantly decreased in the VTA and NAc. These decreases may be ascribed to the morphine-induced, long-lasting release of histamine from nerve terminals in the nuclei. Morphine is known to increase the release of histamine from histaminergic neurons[22,23]. Since treatment with morphine acutely activates histaminergic neurons intensely and persistently, histamine synthesis may not be sufficient to compensate for the release. Perhaps the decrease in the size and number of histaminergic neurons indicates compensatory adaptations to oppose this intense and persistent histamine release. It is likely that the morphological changes in histaminergic neurons resulted from neural injury caused by exposure to morphine. Although the exact mechanisms remain unclear, our data are the first to indicate that endogenous histamine acts as an important neuromo-dulator in the development of the reward process induced by morphine.
We also found that histidine, a precursor of histamine, produced no conditioned place aversion when given alone, but significantly and dose-dependently inhibited the morphine-induced development of CPP. Histidine at a dose of 200 mg/kg significantly reversed the decreased histamine levels induced by morphine in the VTA and NAc (data not shown). Consistent with this finding, the histidine decarboxylase inhibitor, a-fluoromethylhistidine, potentiates morphine-induced CPP in mice[24]. In addition, it is known that the acquisition and expression of CPP behavior requires learning and memory to establish an association between environmental cues and the effective state induced by morphine; cue reactivity in the CPP paradigm is blocked by scopolamine, which impairs learning and memory[25,26]. However, even at the low doses used in the present study, histidine can ameliorate memory deficits in many animal models[27,28]. So it is suggested that the inhibitory action of histidine on morphine-induced CPP in the present study is mainly through the environmental cue reactivity associated with the drug.
Furthermore, bilateral electrolytic lesions in the TM, where histaminergic cell bodies are located, reduced histamine levels in the VTA and NAc as expected, and markedly enhanced the development of the reward-seeking behavior in combination with morphine at a dose that had no appreciable effect on CPP in unlesioned animals. In addition, we also found that HDC knockout mice, in which histamine levels in the brain decreases to almost nil in these mice, were much more sensitive to morphine-induced CPP (data not shown). These results strongly support the idea that endogenous histamine participates in the development of the reward-seeking behavior induced by morphine. Previous studies have also shown that TM lesions with DC or ibotenic acid increase the response rates to lateral hypothalamic self-stimulation[29,30], which suggests that the inactivation of TM histaminergic neurons can result in enhanced reinforcement[31]. On the other hand, neuroactive substances besides histamine are found in the TM, including GABA, glutamate and adenosine[32]. Since electrolytic lesions may non-specifically destroy such neurons or fibers, it was not possible to conclude that the histaminergic neurons were solely responsible for the rewarding effects. However, we found that neither glutamate nor GABA levels in the VTA or NAc changed after TM lesions (Table 2).
We further investigated the effect of histidine as a form of replacement therapy in rats with bilateral TM lesions during morphine-induced development of CPP. The precursor reversed the decreasing histamine levels in the VTA and NAc elicited by TM lesions, and facilitated the development of CPP in parallel (Figure 5). Therefore, we concluded that the decreased brain histamine levels, as a consequence of TM lesions, at least in part potentiate the development of morphine-induce reward-seeking behavior. These results provided further evidence that the morphine-induced reward system may be negatively modulated by endogenous histamine.
The DOPAC/DA ratio is used as an index of DA synthesis and metabolism, and represents dopaminergic neuronal activity[33]. Brain histamine modulates mesolimbic dopaminergic activity from the VTA to the NAc[34]. In this study, we also found that the DOPAC/DA ratios increased in parallel with the decrease of histamine in the VTA and NAc after morphine administration. We found that the TM lesion increased the DOPAC/DA ratio and that histidine reversed this increase. This also suggested that endogenous histamine acts as an inhibitor of the rewarding effect through reducing dopaminergic activity. Decreased brain histamine can weaken the inhibitory effect of dopaminergic activity. Histamine induces hypoactivity and a decreased DOPAC/DA ratio in the striatum[35], while zolantidine, a H2 receptor antagonist, significantly potentiates morphine-induced CPP by increasing the DOPAC/DA ratio[24]. Therefore, DA seems to influence the brain’s reward system in a way which is reciprocal to that of histamine; that is, the brain’s reinforcement mechanism can be activated by DA moving towards more reinforcement and histamine moving it in the opposite direction[36]. An increase in brain histamine levels may attenuate the development of morphine-induced reward-seeking, and its action may be mediated by inhibiting dopaminergic activation in the VTA and NAc. In addition, we found that morphine (1 mg/kg) had no appreciable effect on the DA/DOPAC ratio when given alone, but significantly increased the DA/DOPAC ratio in TM-lesioned rats, which suggests that the activity of the dopaminergic system was facilitated more by morphine when histamine levels were low. So it is proposed that the inhibitory effect on dopaminergic neurons is weakened by a persistent deficiency of histamine, and can further enhance the sensitivity to morphine.
In summary, the present study demonstrates that endogenous histamine is involved in the morphine reward-seeking process and inhibits the development of morphine-induced CPP. The mechanism involves the modulation of dopaminergic activity by endogenous histamine. Therefore, histamine and related compounds may be of value in treating and preventing the development of morphine-induced reward-seeking behavior.
References
- Masukawa Y, Suzuki T, Misawa M. Differential modification of the rewarding effects of methamphetamine and cocaine by opioids and antihistamines. Psychopharmacology (Berl) 1993;111:139-43.
- Houdi AA, Bardo MT, Van Loon GR. Opioid mediation of cocaine-induced hyperactivity and reinforcement. Brain Res 1989;497:195-8.
- Schechter MD, Calcagnetti DJ. Trends in place preference conditioning with a cross-indexed bibliography; 1957–1991. Neurosci Biobehav Rev 1993;17:21-41.
- Schwartz JC, Arrang JM, Garbarg M, Pollard H, Ruat M. Histaminergic transmission in the mammalian brain. Physiol Rev 1991;71:1-51.
- Haas H, Panula P. The role of histamine and the tuberomamillary nucleus in the nervous system. Nat Rev Neurosci 2003;4:121-30.
- Wada H, Inagaki N, Yamatodani A, Watanabe T. Is the histaminergic neuron system a regulatory center for whole-brain activity? Trends Neurosci 1991;14:415-8.
- Panula P, Pirvola U, Auvinen S, Airaksinen MS. Histamine-immunoreactive nerve fibers in the rat brain. Neuroscience 1989;28:585-610.
- Privou C, Knoche A, Hasenohrl RU, Huston JP. The H1- and H2-histamine blockers chlorpheniramine and ranitidine applied to the nucleus basalis magnocellularis region modulate anxiety and reinforcement related processes. Neuropharmacology 1998;37:1019-32.
- Cohn CK, Ball GG, Hirsch J. Histamine: effect on self-stimulation. Science 1973;180:757-8.
- Rassnick S, Kornetsky C. L-Histidine attenuates the effects of pentazocine on rewarding brain-stimulation. Life Sci 1991;48:1729-36.
- Nath C, Patnaik GK, Saxena RC, Gupta MB. Evaluation of inhibitory effect of diphenhydramine on benzodiazepine dependence in rats. Indian J Physiol Pharmacol 1997;41:42-6.
- Halpert AG, Olmstead MC, Beninger RJ. Mechanisms and abuse liability of the anti-histamine dimenhydrinate. Neurosci Biobehav Rev 2002;26:61-7.
- Paxinos G, Watson C. The rat brain in stereotaxic coordinates, 4th ed. San Diego: Academic Press, 1998.
- Jin CL, Yang LX, Wu XH, Li Q, Ding MP, Fan YY, et al. Effects of carnosine on amygdaloid kindled seizures in Sprague-Dawley rats. Neuroscience 2005;135:939-47.
- Doyon WM, York JL, Diaz LM, Samson HH, Czachowski CL, Gonzales RA. Dopamine activity in the nucleus accumbens during consummatory phases of oral ethanol self-administration. Alcohol Clin Exp Res 2003;27:1573-82.
- Moore KE, Demarest KT, Lookingland KJ. Stress, prolactin and hypothalamic dopaminergic neurons. Neuropharmacology 1987;26:801-8.
- Mucha RF, van der Kooy D, O’Shaughnessy M, Bucenieks P. Drug reinforcement studied by the use of place conditioning in rat. Brain Res 1982;243:91-105.
- Suzuki T, Funada M, Narita M, Misawa M, Nagase H. Morphine- induced place preference in the CXBK mouse: characteristics of μ-opioid receptor subtypes. Brain Res 1993;602:45-52.
- Huang EY, Liu TC, Tao PL. Co-administration of dextromethor-phan with morphine attenuates morphine rewarding effect and related dopamine releases at the nucleus accumbens. Naunyn-Schmiedebergs Arch Pharmacol 2003;368:386-92.
- Bals-Kubik R, Ableitner A, Herz A, Shippenberg TS. Neuroanatomical sites mediating the motivational effects of opioids as mapped by the conditioned place preference paradigm in rats. J Pharmacol Exp Ther 1993;264:489-95.
- Olmstead MC, Franklin KB. The development of a conditioned place preference to morphine: effects of microinjections into various CNS sites. Behav Neurosci 1997;111:1324-34.
- Onodera K, Yamatodani A, Watanabe T, Wada H. Neuropharmacology of the histaminergic neuron system in the brain and its relationship with behavioral disoders. Prog Neourobiol 1994;42:685-702.
- Barke KE, Hough LB. Characterization of basal and morphine-induced histamine release in the rat periaqueductal gray. J Neuro-chem 1994;63:238-44.
- Suzuki T, Takamori K, Misawa M, Onodera K. Effects of the histaminergic system on the morphine-induced conditioned place preference in mice. Brain Res 1995;675:195-202.
- Itzhak Y, Martin JL. Scopolamine inhibits cocaine-conditioned but not unconditioned stimulant effects in mice. Psychopharmacology (Berl) 2000;52:216-23.
- Schroeder JP, Packard MG. Posttraining intra-basolateral amygdala scopolamine impairs food- and amphetamine-induced conditioned place preferences. Behav Neurosci 2002;116:922-7.
- Kamei C, Tasaka K. Effect of histamine on memory retrieval in old rats. Biol Pharm Bull 1993;16:128-32.
- Huang YW, Chen Z, Hu WW, Zhang LS, Wu W, Ying LY, et al. Facilitating effect of histamine on spatial memory deficits induced by dizocilpine as evaluated by 8-arm radial maze in SD rats. Acta Pharmacol Sin 2003;24:1270-6.
- Wagner U, Weiler HT, Huston JP. Amplification of rewarding hypothalamic stimulation following a unilateral lesion in the region of the tuberomammillary nucleus. Neuroscience 1993;52:927-32.
- Wagner U, Segura-Torres P, Weiler T, Huston JP. The tubero-mammillary nucleus region as a reinforcement inhibiting substrate: facilitation of ipsihypothalamic self-stimulation by unilateral ibotenic acid lesions. Brain Res 1993;613:269-74.
- Frisch C, Hasenohrl RU, Huston JP. Memory improvement by post-trial injection of lidocaine into the tuberomammillary nucleus, the source of neuronal histamine. Neurobiol Learn Mem 1999;72:69-77.
- Kohler C, Swanson LW, Haglund L, Wu JY. The cytoarchitecture, histochemistry and projections of the tuberomammillary nucleus in the rat. Neuroscience 1985;16:85-110.
- Bannon MJ, Roth RH. Pharmacology of mesocortical dopamine neuron. Pharmacol Rev 1983;35:53-68.
- Fleckenstein AE, Lookingland KJ, Moore KE. Activation of mesolimbic dopaminergic neurons following central administration of histamine is mediated by H1 receptors. Naunyn-Schmiedebergs Arch Pharmacol 1993;347:50-4.
- Chiavegatto S, Nasello AG, Bernardi MM. Histamine and spontaneous motor activity: biphasic changes, receptors involved and participation of the striatal dopamine system. Life Sci 1998;62:1875-88.
- Huston JP, Wagner U, Hasenohrl RU. The tuberomammillary nucleus projections in the control of learning, memory and reinforcement processes: evidence for an inhibitory role. Behav Brain Res 1997;83:97-105.