Melatonin-selenium nanoparticles protects liver against immunological injury induced by bacillus Calmette-Guérin and lipopolysaccharide1
Introduction
Hepatic damage, both acute and chronic, is a common pathology worldwide quite often characterized in its chronic evolution by a progressive process from steatosis to hepatocellular carcinoma through chronic hepatitis, fibrosis, and cirrhosis. The main etiology is represented by viral infections (HBV, HDV, and HCV) and alcohol abuse[1]. The injection of bacillus Calmette-Guérin (BCG) followed by lipopolysaccharide (LPS) is useful for the creation of experimental models of immunological hepatic damage[2]. It is known that Propionibacterium acnes or BCG priming and LPS challenge in mice causes massive liver injury, which consists of priming and eliciting phases. P acnes or BCG priming induces mononuclear cell infiltration into the liver lobules and granuloma formation. The subsequent LPS injection elicits acute and massive hepatic injury, with a host release of reactive oxygen species, nitric oxide (NO) and pro-inflammatory cytokines such as tumor necrosis factor α (TNF-α), interleukin-1β (IL-1β)[3-5].
Melatonin participates in many important physiological processes, including anti-inflammatory[6] and immunoregu-latory[7] processes, as well as acting as an antioxidant[8,9]. It not only functions as a direct antioxidant; that is, as a scavenger of various oxygen free radicals including hydroxyl radical, singlet oxygen, hydrogen peroxide (H2O2) and peroxyl radical, but it also functions as an indirect antioxidant through the enhancement of antioxidant enzyme activities in tissues such as the liver and the brain. Furthermore, melatonin also neutralizes ONOO– and inhibits the production of NO. In addition, our and other published reports have shown that melatonin protects against liver injury through its antioxidant action[10,11].
Selenium (Se) is an essential element for humans and can improve the activity of the seleno-enzyme and glutathione peroxidase (GSH-px), and can also prevent free radicals from damaging cells and tissues in vivo. One of its best-understood functions is that it is present in the active center of GSH-px, an antioxidant enzyme that scavenges various peroxides and protects membrane lipids and macromolecules from oxidative damage[12]. The supplementation of food with selenium is usually limited to selenium-containing com-pounds, such as sodium selenite (Na2SeO3), ebselen, and other organoselenium compounds. However, several studies have been focused on the elemental selenium (Se0). It is currently known that gray and black elemental Se is biologically inert. However, there is one kind of red elemental particulate selenium, observed in several bacteria, which could provide environmental protection from pollution caused by excessive selenium[13]. These elemental selenium particles are formed in the bacteria to detoxify the excess selenium. In the work of Zhang et al[14], it was found that selenium nanoparticles had a higher bioavailability, much less acute toxicity and better scavenging effect compared with organic and other inorganic selenium-containing compounds.
In the present paper, based on the antioxidant and immunoregulatory actions of melatonin and elemental selenium nanoparticles, we combined selenium nanoparticles with melatonin in order to obtain useful materials for medication. Melatonin-selenium nanoparticle (MT-Se), a novel complex, was synthesized by preparing selenium nanoparticles in melatonin medium. In this study, the mechanisms of the protective effects of MT-Se on immunological liver injury induced by BCG plus LPS were investigated.
Materials and methods
Preparation of melatonin-selenium nanoparticle MT-Se was prepared by reducing selenious acid with ascorbic acid in the presence of melatonin. In a synthetic process, the appropriate amount of selenious acid (the water solution of SeO2) was thoroughly mixed with melatonin. Then excessive ascorbic acid was added into the selenious acid/melatonin mixture to initiate redox reaction[15].
After the reaction mixture was mixed for approximately 30 min, the product showing light red was obtained. In the reaction process, melatonin molecules could be strongly absorbed on the surface of nascent selenium seeds. Melatonin not only served as a seed growth controller, but also pre-vented selenium nanoparticles from congregating. Transmission electron microscopy (TEM) images of the product, as shown in Figure 1, were captured on a JEOL-JEM 200CX instrument (JEOL Company, Japan) at an accelerating voltage of 100 kV. From the image, it can be seen that uniform spherical selenium nanoparticles were produced. The final product prepared for medicine application was the complex made up of 95% melatonin, 1% elemental selenium, and 4% ascorbic acid/dehydroascorbic acid. It was dissolved with absolute ethanol and then stabilized and suspended briefly in 0.5% carboxymethylcellulose (CMC) before use.
Materials MT-Se, provided by the Department of Chemistry, Anhui University, was dissolved with absolute ethanol and then stabilized and suspended briefly in 0.5% CMC. Melatonin, purchased from Sigma Chemical (St Louis, MO, USA), was suspended in 0.5% CMC with absolute ethanol (≤0.01%, v/v) and stored at -20 ºC. LPS from Escherichia coli were obtained from Sigma Chemical. Commercial kits used for determining lipid peroxidation (TBRAS), GSH-px and superoxide dismutase (SOD) activity were obtained from the Jiancheng Institute of Biotechnology (Nanjing, China). Other chemicals used in these experiments were analytical grade from commercial sources.
Animals Male Kunming mice (20±2 g), obtained from the Animal Department of Anhui Medical University, were maintained on a 12 h light/12 h dark cycle from 6:00 AM to 18:00 PM under a regulated environment (20±1 ºC). Animals were housed in plastic cages with free access to food and water. All procedures followed the guidelines for human treatment of animals set by the Association of Laboratory Animal Sciences and the Center for Laboratory Animal Sciences at Anhui Medical University.
Establishment of acute liver injury model BCG (viable bacilli), 2.5 mg suspended in 0.2 mL saline, was injected through the tail vein in mice, and 10 d later they were injected with 7.5 µg LPS dissolved in 0.2 mL saline. The mice were anesthetized with ether then killed 16 h after LPS injection by cervical dislocation. The trunk blood was collected into heparinized tubes (50 kU/L) and centrifuged (1500×g, 10 min, 4 ºC). Serum was aspirated and stored at -70 ºC until assayed as described below. The liver was also removed and stored at -70 ºC until required[2,10].
Drug treatment Seven groups of mice, 10 in each, were arranged as follows: (1) normal untreated mice; (2) model mice; (3) model mice treated with different drugs: (a) three MT-Se groups (5, 10, and 20 mg/kg respectively); (b) bifendate group (100 mg/kg). In the treatment groups, different drugs were administered orally by gastric intubation 10 d prior to LPS injection. The mice in normal and model control groups were fed the same volume of vehicle only.
Measurement of serum alanine aminotransferase and aspartate aminotransferase Serum alanine aminotransferase (ALT) and aspartate aminotransferase (AST) were determined using commercial kits produced by Jiancheng Institute of Biotechnology (Nanjing, China). Their activities were expressed as an international unit (U/L).
Histological analysis Formalin-fixed specimens were embedded in paraffin and stained with hematoxylin and eosin for conventional morphological evaluation. After decapitation of rats, small hepatic specimens were placed in 10% (v/v) formalin solution and processed routinely by embedding in paraffin. Tissue sections (4–5 µm) were stained with hematoxylin and eosin and examined under light microscope (Olympus IX70, Japan). An experienced histologist, who was unaware of the treatment conditions, made histological assessments.
Measurement of malondialdehyde, SOD, and GSH-px in liver homogenate Lipid peroxidation production in the liver tissue was determined by measuring thiobarbituric acid-reactive substances (TBARS)[16]. TBARS were measured using the thiobarbituric acid method, with 1,1,3,3-tetra-methoxypropane as a standard. Hepatic protein was assayed by the method of Lowry et al using bovine serum albumin as a standard[17]. The amount of TBARS was expressed as nmol malondialdehyde (MDA)/mg protein. The assay for total SOD was based on its ability to inhibit the oxidation of oxymine by the xanthine-xanthine oxidase system[18]. Results were expressed as U per microgram protein. The activity of GSH-px was determined by quantifying the rate of H2O2-induced oxidation of GSH to oxidized glutathione (GSSH). A yellow product with absorbance at 412 nm was formed as GSH reacted with dithionitrobenzoic acid[19]. One unit of GSH-px was defined as the amount that reduced the level of GSH by 1 µmol/L in 1 min/mg protein.
Measurement of NO in serum and liver NO in the serum and the liver was measured by a microplate assay using Griess reagent, which produces a chromophore with the nitrite[20]. Briefly, 100 µL of supernatants were removed and incubated with 100 µL of Griess reagent (1% sulfanilamide and 0.1% N-1-naphthylethylenediamine dihydrochloride in 2.5% phosphoric acid) in a 96-well plate. The plate was incubated for 10 min at room temperature. Nitrite production was quantified spectrophotometrically using an automated colorimetric procedure. Absorbance at 540 nm was measured using a microplate reader (318MC, Shanghai Sanco). The nitrite concentration was calculated by comparing samples with standard solutions of sodium nitrite produced in the culture medium. All samples were assayed in triplicate. Results were expressed as µmol/L.
Splenocytes proliferation assay Mice were killed by cervical dislocation. Spleens were removed in sterile conditions and splenocytes were collected. Then the cells were suspended in RPMI-1640 medium at a concentration of 1×107 cell/L. The cell suspension (100 µL) and concanavalin A (ConA) (100 µL with final concentration of 3 mg/L) or LPS (100 µL with final concentration of 4 mg/L) were seeded to a 96-well culture plate simultaneously. Triplicates were designed. The cultures were incubated at 37 ºC in an atmosphere of 5% CO2 for 48 h. Two hours before completion, 10 µL of MTT (5 g/L) was added to each well. The absorbance was measured on an EJ301 ELISA Microwell Reader (Wuxi Keda, China) at 570 nm. The results were described as the average of triplicate A.
Preparation of peritoneal macrophages and induction of TNF-α and IL-1β Mice PMΦ were collected in D-Hanks’ medium by routine method. Then PMΦ was resuspended in RPMI-1640 medium at 1×109 cell/L and the cell suspension was seeded into a 24-well culture plate, 1 mL per well. After incubation at 37 ºC for 2 h in 5% CO2 atmosphere, supernatants were removed and the adherent cells were washed 3 times with Hanks’ medium, which contained 5% neonatal bovine serum. Thus, the monolayer of PMΦ was obtained. LPS, with a final concentration of 4 mg/L, was added to each well and RPMI-1640 was also added to make a final volume per well of up to 1 mL. The plate was then incubated at 37 ºC in air with 5% CO2 for 48 h. After being centrifuged (500×g, 10 min), all the supernatants containing extracellular TNF-α and IL-1β were collected and stored at -20 ºC until assay.
Measurement of TNF-α and IL-1β in liver and culture supernatants The TNF-α and IL-1β levels in the liver homogenate and culture supernatants were determined using rat ELISA kits. Their levels were expressed as ng/L.
Statistical analysis All values are presented as mean±SD. A two-tailed, non-paired, Student’s t-test was used to evaluate differences between means. Statistical significance was set at P<0.05.
Results
Effect of MT-Se on serum ALT and AST concentration The model group (treated with BCG plus LPS) manifested severe liver damage with an elevation of serum amino-transferase, compared with the normal group. In the group of mice treated with MT-Se (5, 10, and 20 mg/kg), serum levels of liver enzymes were significantly decreased as compared with BCG plus LPS-treated mice. Similarly, bifendate (100 mg/kg) reduced the levels of liver enzymes as opposed to BCG plus LPS-treated mice (Table 1).
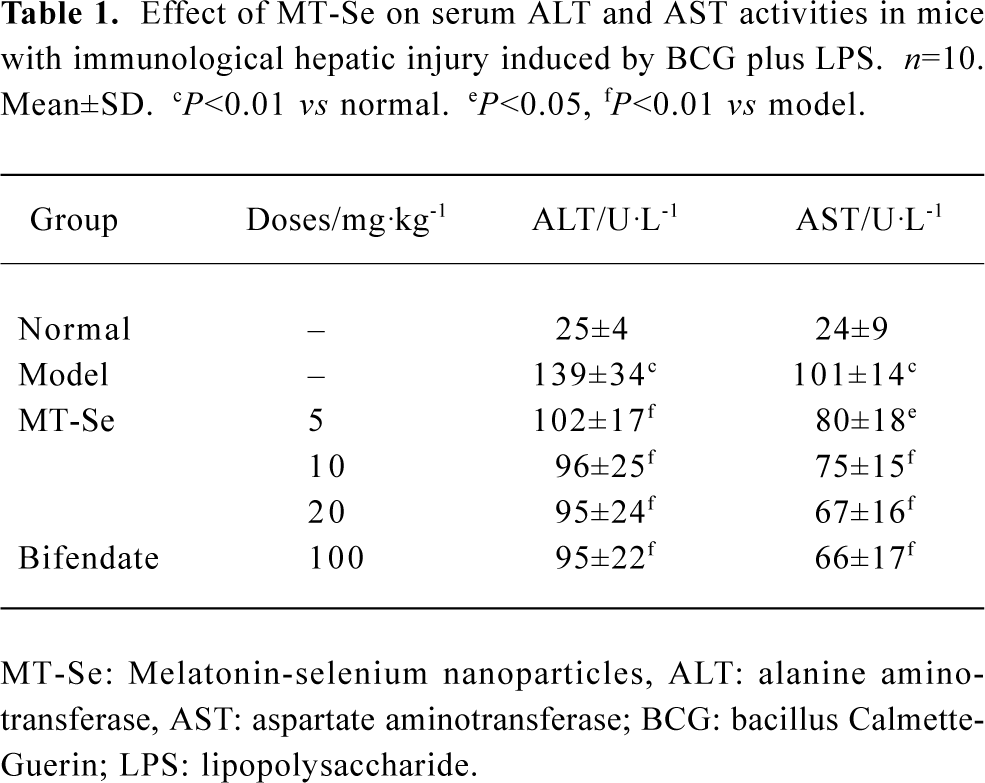
Full table
Effect of MT-Se on liver histology No histological abnormalities were observed in normal mice. There was severe necrosis of hepatocytes with marked mononuclear infil-tration, seen after LPS injection in the BCG-primed mice. In the MT-Se (5, 10, and 20 mg/kg) treated groups, the area and extent of necrosis was attenuated and the immigration of inflammatory cells was reduced (Figure 2).
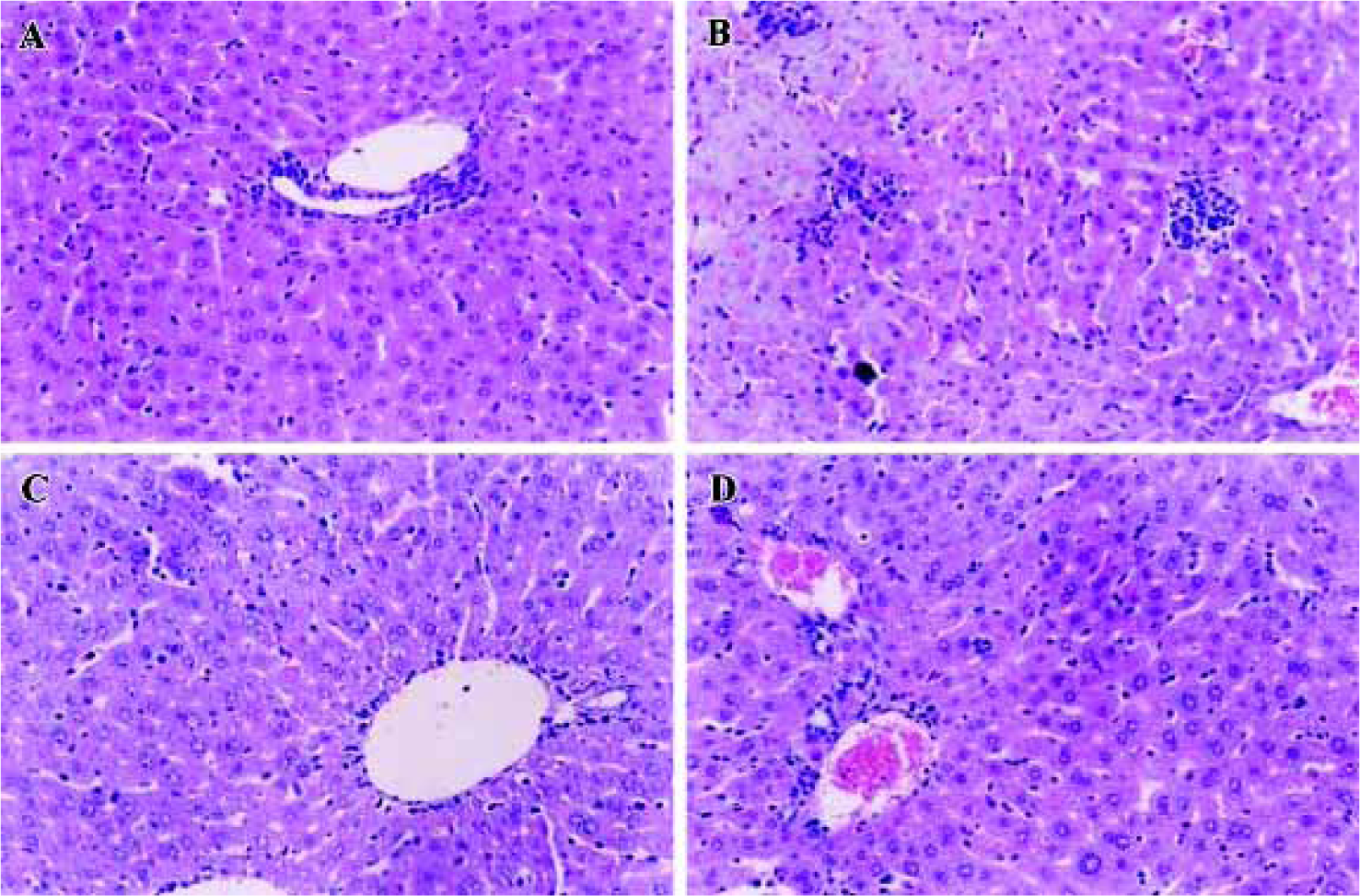
Effect of MT-Se on liver homogenate MDA content and SOD, GSH-px activities Injection of LPS after BCG priming in mice caused a significant elevation in MDA levels in the liver tissue. MT-Se (5, 10 and 20 mg/kg) reduced the elevation of MDA levels in the liver. The activities of antioxidant enzymes including GSH-px and SOD were significantly inhibited in the liver tissues of mice injected with LPS after BCG priming. Treatment with MT-Se (10 and 20 mg/kg) increased the activity of GSH-px and MT-Se at doses of 10 and 20 mg/kg elevated the activity of SOD (Table 2).
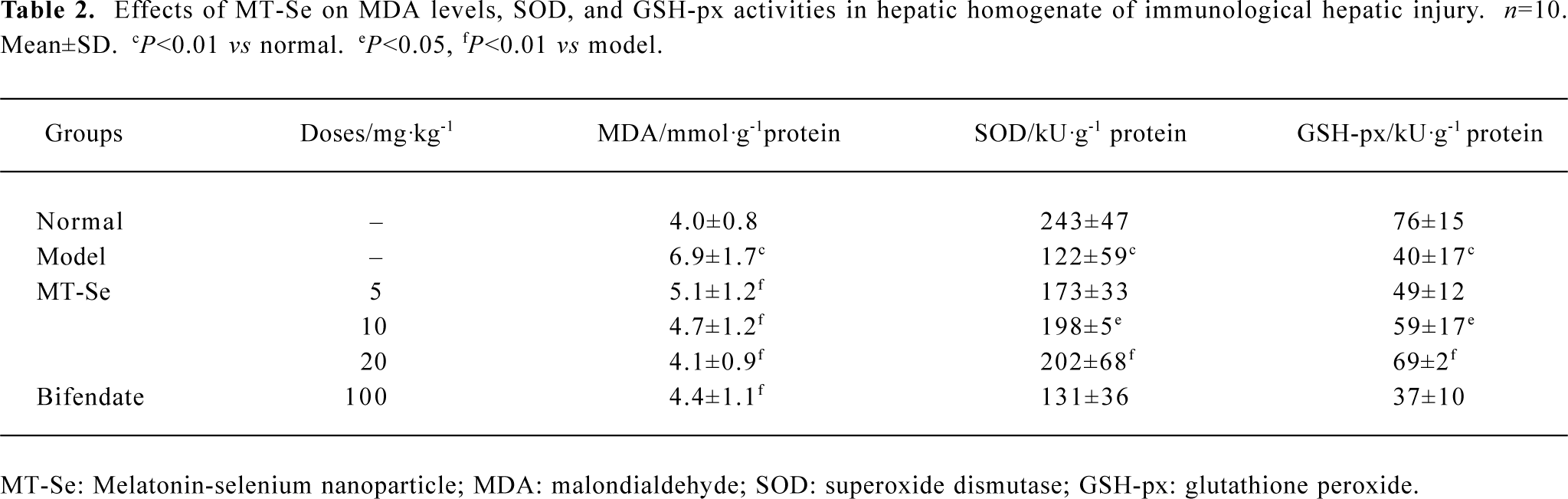
Full table
Effect of MT-Se on serum and liver levels of NO The levels of NO in serum and liver tissue were significantly increased in BCG-primed mice challenged with LPS. MT-Se (5, 10, and 20 mg/kg) reduced the elevation of NO levels in serum and liver tissue (Figure 3).
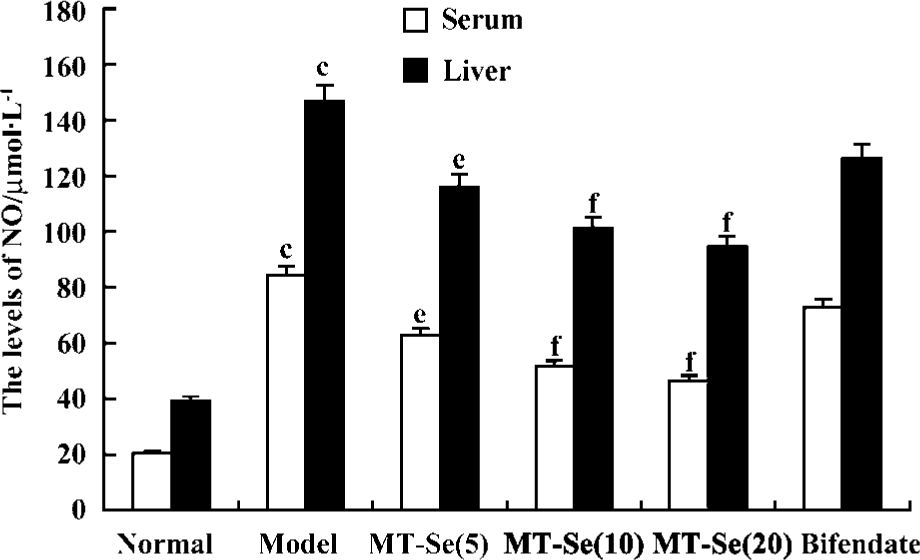
Effect of MT-Se on TNF-α and IL-1β concentration in the liver As shown in Table 3, when mice were first injected with BCG and then challenged with LPS, the proinflammatory cytokines, such as TNF-α and IL-1β, were significantly elevated. MT-Se (5, 10, and 20 mg/kg) decreased the levels of TNF-α and IL-1β in a liver homogenate. Bifendate had no effect on TNF-α and IL-1β (Table 3).
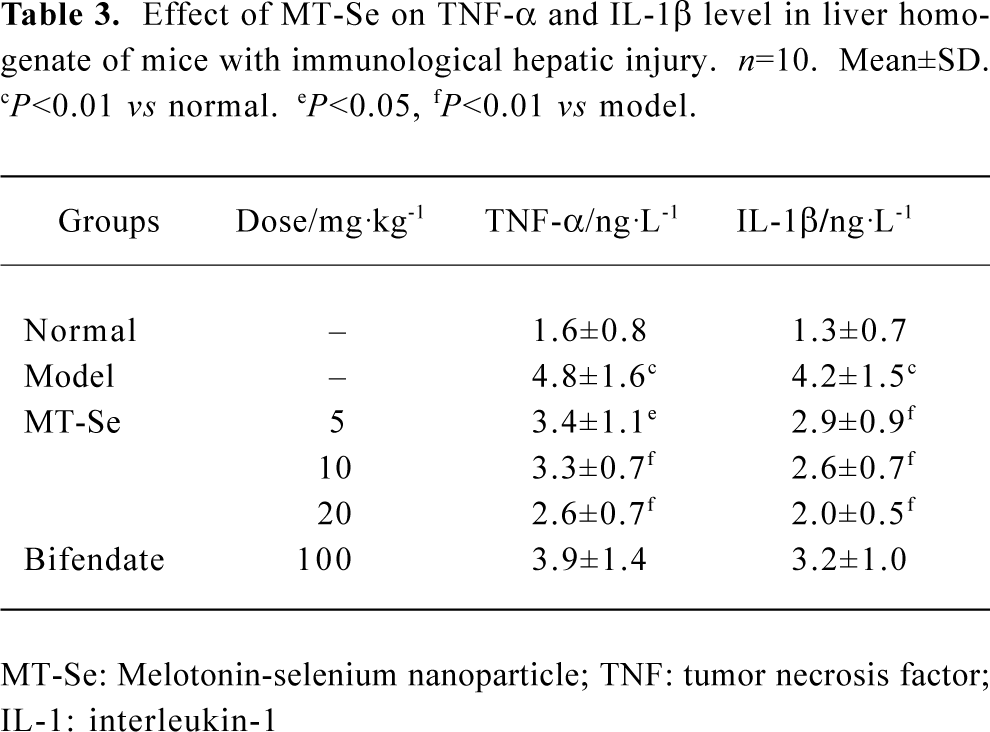
Full table
Effect of MT-Se on splenocyte proliferation in mice with immunological liver injury Treatment with MT-Se suppressed the accentuation of splenocyte proliferation induced by LPS or ConA (Figure 4).
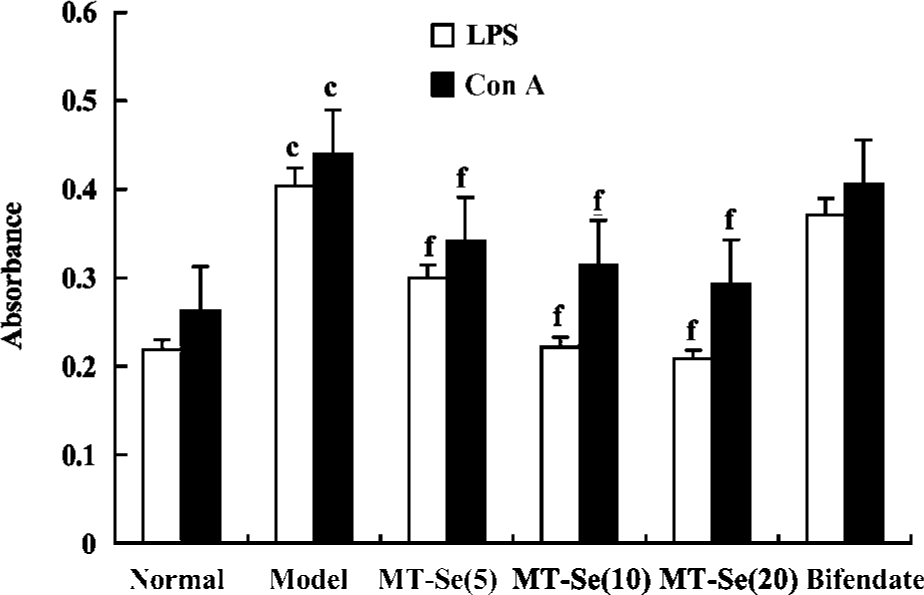
Effect of MT-Se on production of TNF-α and IL-1β by PMΦ The levels of TNF-α and IL-1β in culture supernatants of PMΦ were significantly increased in immunological liver injury mice. MT-Se (5, 10, and 20 mg/kg) inhibited the production of TNF-α and IL-1β (Table 4).
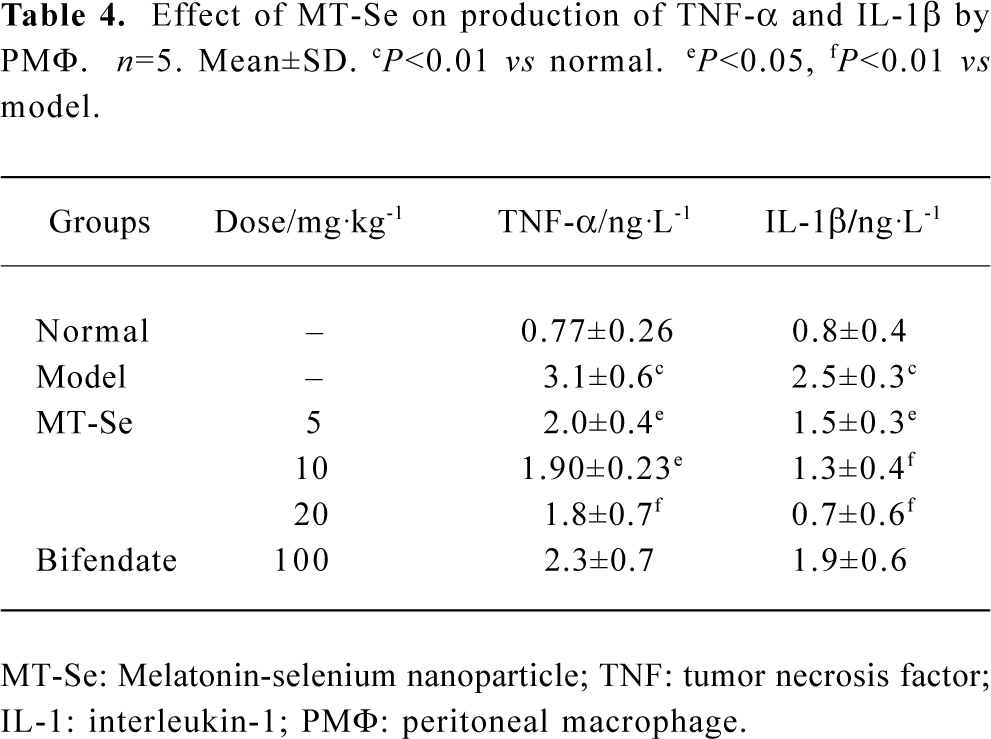
Full table
Discussion
The findings of the present study showed that MT-Se, a new chemical compound that is composed of melatonin and red elemental selenium particles, prevented the development of immunological liver injury induced by BCG plus LPS. It markedly reduced the severe liver injury in mice as demonstrated by a significant reduction in the serum transaminase levels and amelioration of severe hepatic pathological abno-rmalities. Meanwhile, MT-Se decreased MDA content and NO levels, and increased the antioxidant capacity, including SOD and GSH-px activity. In addition, MT-Se played an immunoregulatory role in decreasing proinflammatory cytokines and inhibiting splenocyte proliferation. We propose that oxidative stress and proinflammatory cytokine overproduction imposed on the liver play an important role in the pathogenesis of hepatic damage, and these results are attenuated by the antioxidant and immunoregulatory activity of MT-Se.
The current results confirmed that MT-Se’s protection against hepatic injury was associated with the antioxidant property of melatonin and selenium. Melatonin combined with selenium could induce synergistic effects, indicated by improved free radical scavenging activity of MT-Se. Selenium, an integral part and located at the catalytic site of GSH-px, is now known to be of fundamental importance to human health[21]. Recent evidence has shown that treatment with selenium and other antioxidants results in positive clinical responses in various liver diseases associated with increased oxidative damage[22]. GSH-px and some seleno-compounds such as ebselen have also been shown to protect against hepatic injury[23,24]. Selenium also appears to be protective for individuals infected with the hepatitis virus (B or C) against the progression of the condition to liver cancer[25]. These effects may prove selenium to be beneficial as an adjuvant therapy for liver disease. However, it must be remembered that selenium is a toxic mineral with a fairly small therapeutic window. Seleno-compounds, especially inorganic selenium, could be hepatotoxic and teratogenic in animals and humans. In some sensitive individuals, the maximum safe dietary intake may be as low as 600 µg per day. It would therefore seem prudent to restrict adult intake from all sources to an upper limit of 400–450 µg/day as recommended by several expert panels[26]. Although the toxic effect of elemental selenium nanoparticles is less, the selenium contents percentage in MT-Se is only 1% and the dose of selenium less than 200 µg in our experimental design. The current study confirmed that selenium at doses of 50 to 200 μg reinforced the endogenous anti-oxidative systems in comparison with melatonin or selenium alone; suggested by MT-Se blunting of lipid peroxidation and increased GSH-px activity.
Melatonin is also well known to be a multi-faceted free radical scavenger and antioxidant[8,9]. Previous studies have shown that melatonin could protect against several models of liver injury by inhibiting oxidative and nitrosative damage. Calvo et al found that melatonin protected against alpha-naphthylisothiocyanate (ANIT)-induced liver injury with cholestasis in rats, and suggested that this protective effect was a result of its antioxidative properties and above all to its capacity to inhibit liver neutrophil infiltration, a critical factor in the pathogenesis of ANIT-induced liver injury[27]. Melatonin at high doses (10–100 mg/kg) could also dose-dependently reduce liver lipid peroxide content in CCl4-treated rats. This indicates that melatonin exerts a therapeutic effect on CCl4-induced acute liver injury in rats, possibly through its antioxidant action[28]. Melatonin plays a cytoprotective role in the liver affected by ischemia and reperfusion through its ability to prevent hepatic malfunction and inhibit the generation of free radicals and accumulation of neutrophils in the damaged hepatic tissue[29]. Moreover, melatonin appeared to be significantly more potent than N-acetylcysteine in reversing the oxidative damage induced by ischemia and reperfusion[30]. In bile duct ligated rats, melatonin was much more effective than vitamin E in reducing liver injury and oxidative stress[31]. Acetaminophen at high doses can cause fulminant hepatic necrosis and nephrotoxic effects, and melatonin can reverse these effects with its higher efficacy in scavenging various free radicals and also because of its ability in stimulating the antioxidant enzymes[32]. In our previous study, we also observed that pretreatment with melatonin had a protective effect on immunological hepatic damage induced by BCG and LPS. In this study, it is confirmed that MT-Se also had a hepatic protective effect through the synergistic antioxidant effect of melatonin and selenium.
As is well known, TNF-α is considered to be a common early effector molecule for liver injury, in addition to its direct cytotoxic effects, this cytokine is able to induce chemo-kines, macrophage chemotactic protein-1, and adhesive molecules (vascular-cell adhesion molecule-1), which are key to inflammation and consequent liver damage. The prevention of liver injury has been observed upon neutralization of TNF-α with anti-TNF-α antibody, prevention of translation of primary RNA transcript of TNF-α by antisense oligonucleotide and interaction of TNF-α with soluble TNF-α receptors. Although IL-1 itself does not damage the liver, its elevation could stimulate inflammatory cells to excrete many other cytokines including TNF-α, IL-6, and IL-8. Our results suggest that the elevation of inflammatory cytokines, including TNF-α and IL-1β, in the liver tissue and PMΦ cell culture supernatants are contributing to the mechanisms of immunological liver injury. MT-Se decreased TNF-α and IL-1β levels in the liver inhibiting their production by PMΦ. These results are consistent with the literature suggesting mela-tonin’s immunosuppressive effect on the production of inflammatory cytokines. Shin et al[33] found that low levels of Bacillus anthracis were known to induce the release of cyto-kines such as TNF-α, and thereby exposure of melatonin (1×10-7−1×10-6 mol) to anthrax lethal toxin-treated macrophages also decreased the release of TNF-α to the extracellular medium as compared with the control. Sacco et al[34] found that the administration of melatonin to mice (5 mg/kg, sc, 30 min before or simultaneously with LPS) inhibited serum TNF-α levels by 50%–80% and improved the survival of mice treated with a lethal dose of LPS. It was also previously reported that melatonin had a protective role in LPS-induced septic shock by suppressing proinflammatory cytokines, prostaglandins and NO production[35]. Thus, the present study revealed that MT-Se had an immunoregulatory effect on immunological liver injury in mice by inhibiting proinflammatory cytokine production. Moreover, in our in vivo experiment, MT-Se suppressed the proliferation of spleen cells induced by ConA or LPS. These findings suggest that MT-Se may alleviate immunological liver injury not only through inhibiting the activation of PMΦ, but also by inducing the dysfunction of activated lymphocytes.
In summary, the results obtained in the present study indicate that the mode of MT-Se’s hepatic protective action is, at least in part, related to its antioxidant properties, such as inhibiting lipid peroxidation and NO production, as well as increasing the antioxidant enzyme capacity. Furthermore, we found that it appeared that MT-Se had an immunoregulatory effect on inhibiting proinflammatory cytokines and activated lymphocytes. MT-Se, a novel complex, may be beneficial as a valuable drug for protection against liver injury. However, further investigation is required to clarify the detailed mechanism of the therapeutic effect of MT-Se on liver injury.
References
- Hoek JB, Pastorino JG. Ethanol, oxidative stress, and cytokine-induced liver cell injury. Alcohol 2002;27:63-8.
- Wang GS, Liu GT. Influences of Kupffer cell stimulation and suppression on immunological liver injury in mice. Acta Pharmacol Sin 1997;18:173-6.
- Guler R, Olleros ML, Vesin D, Parapanov R, Vesin C, Kantengwa S, et al. Inhibition of inducible nitric oxide synthase protects against liver injury induced by mycobacterial infection and endotoxins. J Hepatol 2004;41:773-81.
- Nagakawa J, Hishinuma I, Hirota K, Miyamoto K, Yamanaka T, Tsukidate K, et al. Involvement of tumor necrosis factor-α in the pathogenesis of activated macrophage mediated hepatitis in mice. Gastroenterology 1990;99:758-65.
- Tsuji H, Harada A, Mukaida N, Nakanuma Y, Bluethmann H, Kaneko S, et al. Tumor necrosis factor receptor p55 is essential for intrahepatic granuloma formation and hepatocellular apopto-sis in a murine model of bacterium-induced fulminant hepatitis. Infect Immun 1997;65:1892-8.
- Cuzzocrea S, Reiter RJ. Pharmacological actions of melatonin in acute and chronic inflammation. Curr Top Med Chem 2002;2:153-65.
- Guerrero JM, Reiter RJ. Melatonin-immune system relationships. Curr Top Med Chem 2002;2:167-79.
- Reiter RJ, Tan DX, Mayo JC, Sainz RM, Leon J, Czarnocki Z. Melatonin as an antioxidant: biochemical mechanisms and pathophysiological implications in humans. Acta Biochim Pol 2003;50:1129-46.
- Rodriguez C, Mayo JC, Sainz RM, Antolin I, Herrera F, Martin V, et al. Regulation of antioxidant enzymes: a significant role for melatonin. J Pineal Res 2004;36:1-9.
- Wang H, Wei W, Shen YX, Dong C, Zhang LL, Wang NP, et al. Protective effect of melatonin against liver injury in mice induced by Bacillus Calmette-Guerin plus lipopolysaccharide. World J Gastroenterol 2004;10:2690-6.
- Bruck R, Aeed H, Avni Y, Shirin H, Matas Z, Shahmurov M, et al. Melatonin inhibits nuclear factor kappa B activation and oxidative stress and protects against thioacetamide induced liver damage in rats. J Hepatol 2004;40:86-93.
- Jozanov-Stankov O, Demajo M, Djujic I, Mandic M. Selenium intake as a modulator of responsiveness to oxidative stress. J Environ Pathol Toxicol Oncol 1998;17:251-7.
- Tomei FA, Barton LL, Semanski CL. Transformation of selenate and selenite to elemental selenium by Desulfovibrio desulfuricans. J Ind Microbiol 1995;14:329-36.
- Zhang JS, Gao XY, Zhang LD, Bao YP. Biological effects of a nano red elemental selenium. Biofactors 2001;15:27-38.
- Mees DR, Pysto W, Tarcha PJ. Formation of selenium colloids using sodium ascorbate as the reducing agent. J Colloid Interface Sci 1995;170:254-90.
- Shechter I, Fogelman AM, Haberland ME, Seager J, Hokom M, Edwards PA. Effect of polyunsaturated fatty acids and antioxidants on lipid peroxidation in tissue cultures. J Lipid Res 1981;22:63-9.
- Lowry OH, Rosebrough NJ, Farr AL, Randall RJ. Protein measurement with the Folin-phenol reagent. J Biol Chem 1951;193:265-73.
- Sykes JA, McCormack FX Jr, O’Brien TJ. A preliminary study of the superoxide dismutase content of some human tumors. Cancer Res 1978;38:2759-62.
- Paglia DE, Valentine WN. Studies on the quantitative and qualitative characterization of erythrocyte glutathione peroxidase. J Lab Clin Med 1967;70:58-169.
- Kiechle FL, Malinski T. Nitric oxide. Biochemistry, pathophysiology, and detection. Am J Clin Pathol 1993;100:567-75.
- Rotruck JT, Pope AL, Ganther HE, Swanson AB, Hafeman DG, Hoekstra WG. Selenium: biochemical role as a component of glutathione peroxidase. Science 1973;179:588-90.
- Sakaguchi S, Iizuka Y, Furusawa S, Tanaka Y, Takayanagi M, Takayanagi Y. Roles of selenium in endotoxin-induced lipid peroxidation in the rats liver and in nitric oxide production in J774A.1 cells. Toxicol Lett 2000;118:69-77.
- Ozdil S, Bolkent S, Yanardag R, Arda-Pirincci P. Protective effects of ascorbic acid, dl-alpha-tocopherol acetate, and sodium selenate on ethanol-induced liver damage of rats. Biol Trace Elem Res 2004;97:149-62.
- Kono H, Arteel GE, Rusyn I, Sies H, Thurman RG. Ebselen prevents early alcohol-induced liver injury in rats. Free Radic Biol Med 2001;30:403-11.
- Yu MW, Horng IS, Hsu KH, Chiang YC, Liaw YF, Chen CJ. Plasma selenium levels and the risk of hepatocellular carcinoma among men with chronic hepatitis virus infection. Am J Epidemiol 1999;150:367-74.
- Rayman MP. The importance of selenium to human health. Lancet 2000;356:233-41.
- Calvo JR, Reiter RJ, Garcia JJ, Ortiz GG, Tan DX, Karbownik M. Characterization of the protective effects of melatonin and related indoles against alpha-naphthylisothiocyanate-induced liver injury in rats. J Cell Biochem 2001;80:461-70.
- Ohta Y, Kongo-Nishimura M, Matsura T, Yamada K, Kitagawa A, Kishikawa T. Melatonin prevents disruption of hepatic reactive oxygen species metabolism in rats treated with carbon tetrachloride. J Pineal Res 2004;36:10-7.
- Okatani Y, Wakatsuki A, Reiter RJ, Enzan H, Miyahara Y. Protective effect of melatonin against mitochondrial injury induced by ischemia and reperfusion of rat liver. Eur J Pharmacol 2003;469:145-52.
- Sener G, Tosun O, Sehirli AO, Kacmaz A, Arbak S, Ersoy Y, et al. Melatonin and N-acetylcysteine have beneficial effects during hepatic ischemia and reperfusion. Life Sci 2003;72:2707-18.
- Ohta Y, Kongo M, Kishikawa T. Melatonin exerts a therapeutic effect on cholestatic liver injury in rats with bile duct ligation. J Pineal Res 2003;34:119-26.
- Sener G, Sehirli AO, Ayanoglu-Dulger G. Protective effects of melatonin, vitamin E and N-acetylcysteine against acetaminophen toxicity in mice: a comparative study. J Pineal Res 2003;35:61-8.
- Shin S, Hur GH, Kim YB, Yeon GB, Park KJ, Park YM, et al. Dehydroepiandrosterone and melatonin prevent Bacillus anthracis lethal toxin-induced TNF production in macrophages. Cell Biology Toxicol 2000;16:165-74.
- Sacco S, Aquilini L, Ghezzi P, Pinza M, Guglielmotti A. Mechanism of the inhibitory effect of melatonin on tumor necrosis factor production in vivo and in vitro. Eur J Pharmacol 1998;343:249-55.
- Maestroni G.J. Melatonin as a therapeutic agent in experimental endotoxic shock. J Pineal Res 1996;20:84-9.