Effect of resveratrol on L-type calcium current in rat ventricular myocytes
Introduction
Resveratrol (trans-3,4',5-trihydroxystilbene) is a common phytoalexin that is found in some edible materials, including grape skins, peanuts, and red wine. It has been reported to have a variety of estrogenic, anti-inflammatory, anti-platelet, and anti-carcinogenic effects[1–4]. There is accumulating evidence that resveratrol might serve as a cardioprotective agent. It is possible that resveratrol might protect the heart from ischemia-reperfusion injury, decrease plasma triglyceride and cholesterol accumulation in the aorta and prevent atherosclerosis[5,6]. Moreover, resveratrol has been found to relax the coronary arteries[7,8]. Recently, we found that resveratrol decreased the amplitude of action potential (APA), overshoot (OS) and maximal velocity of phase 0 depolarization (Vmax) in partially depolarized papillary muscles[9]. Moreover, resveratrol inhibited delayed afterdepolarization (DAD) and triggered activity (TA) induced by ouabain in guinea pig papillary muscles[10]. These effects were likely due to a decrease in calcium influx. In addition, Dobrydneva et al found that resveratrol could directly block calcium channels and inhibit calcium influx in human platelets[11]. However, no study regarding the effect of resveratrol on ICa-L in ventricular myocytes has been reported. The present study was undertaken to observe the effect of resveratrol on L-type calcium channels and to investigate the mechanism by which it exerts this effect in rat ventricular myocytes using the whole-cell patch-clamp recording technique.
Materials and methods
Isolation of ventricular myocytes Single ventricular myocytes were obtained by using the modified enzymatic dissociation technique[12]. Male Sprague-Dawley rats (340±40 g, grade II, Certificate N
Measurement of Ca2+ current Isolated ventricular myocytes were placed in an experimental chamber (0.4 mL) mounted on the stage of an inverted microscope (CK2, Olympus). After being settled to the bottom of the chamber, cells were superfused with external solution for 10 min at a rate of 2–3 mL/min at 25 °C. Transmembrane currents were recorded with an Axopatch amplifier (200 B; Axon Instruments). Glass microelectrodes were made using a microelectrode puller (PB-7; Narishige, Japan) by 2-stage pulling and had a resistance of 2.0–4.0 MΩ when filled with electrode internal solution. Only rod-shaped cells with visible cross-striations were used for experiments. Liquid junction potential between the pipette solution and external solution was corrected after the pipette tip was placed into the external solution. After gigaseal formation, the membrane was ruptured with a gentle suction to obtain the whole cell voltage-clamp configuration. To minimize the duration of capacitive current, membrane capacitance and series resistance were compensated after membrane rupture. The external solution was changed to Na+-free solution in which Na+ was replaced by equimolar tetraethylammonium chloride (TEA-Cl). Na+ current was also inactivated at the holding potential (Eh) of -50 mV and blocked by tetrodotoxin (TTX; 2×10-6 mol/L). K+ current was suppressed by substituting intracellular K+ with Cs+. Computer-generated voltage pulses were programmed using pCLAMP 6.0 software (Axon Instruments). Online acquired data were stored on the hard disk of a microcom-puter. All experiments were carried out at room temperature (22–24 °C).
Solutions and drugs Resveratrol, collagenase type II, taurine, tetraethylammonium chloride (TEA-Cl), N-2-hydroxyethylpiperazine-N'-2-ethanesulfonic acid (HEPES), egtazic acid, CsOH, CsCl, and MgATP were purchased from Sigma. TTX was purchased from the Hebei Ocean Product Institute of China (Qinhuangdao City, Hebei Province, China).
The Ca2+-free Tyrode’s solution contained (in mmol/L) NaCl 135, KCl 5.4, MgSO4 1.0, Na H2PO4 0.33, glucose 5, HEPES 10, the pH was adjusted to 7.4 with NaOH. The KB solution contained (in mmol/L) KOH 80, KCl 40, NaH2PO4 25, MgSO4 3, glutamic acid 50, taurine 20, ethyleneglycol-bis(β-amino-ethyl ether)-N,N,N',N'-tetraacetic acid (EGTA) 1, HEPES 10, and glucose 10, the pH was adjusted to 7.4 with KOH. The electrode internal solution for whole-cell recording was composed of (in mmol/L): MgATP 3, CsCl 140, HEPES 10, egtazic acid 10, the pH was adjusted to 7.2 with CsOH. The external solution was composed of (in mmol/L): TEA-Cl 140, MgCl2 2.0, CaCl2 1.5, glucose 10, HEPES 10, TTX 0.002; and was gassed with 100% O2, and the pH was adjusted to 7.3–7.4 with TEAOH. Resveratrol was dissolved in dimethylsulfoxide and diluted in the external solution to achieve concentrations of 10, 20, and 40 µmol/L. The same amount of dimethylsulfoxide was also added to the normal external solution as a control. No change was observed during perfusion with the control solution.
Statistical analysis Data are expressed as mean±SD. Statistical analysis was performed using the t-test and one-way ANOVA. P<0.05 was considered statistically significant.
Results
Effect of resveratrol on L-type calcium current L-type Ca2+ current in rat ventricular myocytes was evoked by using a depolarizing step pulse from a holding potential (Eh) of -50 mV to 0 mV at a frequency of 0.1 Hz. The step pulse dura-tion was 350 ms. The rundown of ICa-L was minimized by adding Mg-ATP (3 mmol/L) and egtazic acid (10 mmol/L)[13]. Resveratrol at concentrations of 10, 20, and 40 µmol/L inhibited the peak amplitude of ICa-L in a concentration-dependent manner (Figure 1). The inhibitory effect of resveratrol disappeared after 15 min washout with control solution.
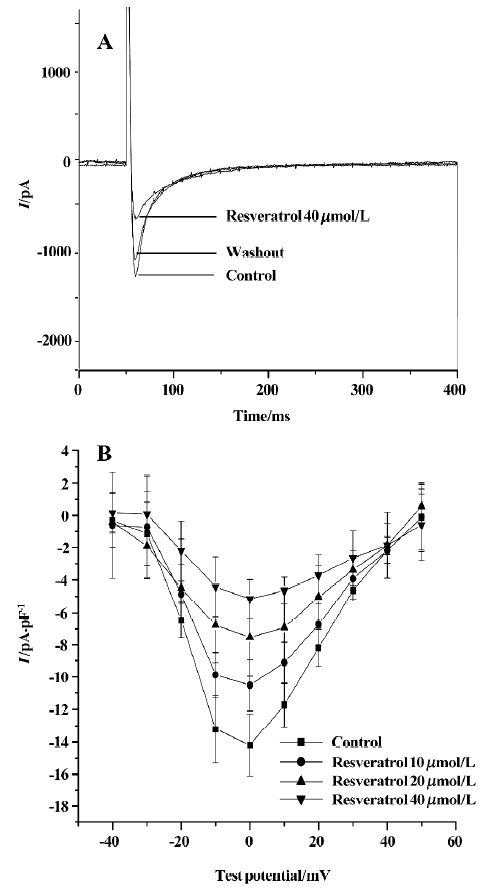
Effect of resveratrol on current-voltage relationship of ICa-L The current-voltage (I–V) curve of L-type Ca2+ current was obtained by using a number of depolarizing step pulses (350 ms) from an Eh of -50 mV to test potentials between -40 mV and 50 mV in 10 mV increments. The pulse frequency was 0.1 Hz. ICa-L was activated at -30 mV and the peak amplitude occurred at a potential of 0 mV. Resveratrol at concentrations of 10, 20, and 40 µmol/L shifted the I–V curve upwards, and decreased the peak amplitude of ICa-L from -14.2±1.5 pA/pF to -10.5±1.5 pA/pF (n=6 cells from 5 hearts; P<0.05), –7.5±2.4 pA/pF (P<0.01) and -5.2±1.2 pA/pF (P<0.01), respectively (Figure 1).
Effects of resveratrol on activation of ICa-L Steady-state activation of L-type calcium channels was obtained by using depolarizing pulses from an Eh of -50 mV to the test potential of +50 mV in 10 mV increments for 350 ms. The pulse frequency was 0.1 Hz. The activation curves were fitted according to the Boltzmann equation: I/Imax=1/{1+EXP[(V-V0.5)/κ]}. V0.5 is the midpoint voltage of the activation functions, and κ is the Boltzmann slope parameter for activation. Resveratrol at a concentration of 40 µmol/L shifted the half-activation potential (V0.5) from 19.4±0.4 mV to -15.4±1.9 mV, and the slope parameter (κ) from 5.4±0.8 mV to 3.8±0.6 mV (n=6 cells from 5 hearts; P<0.05; Figure 2).
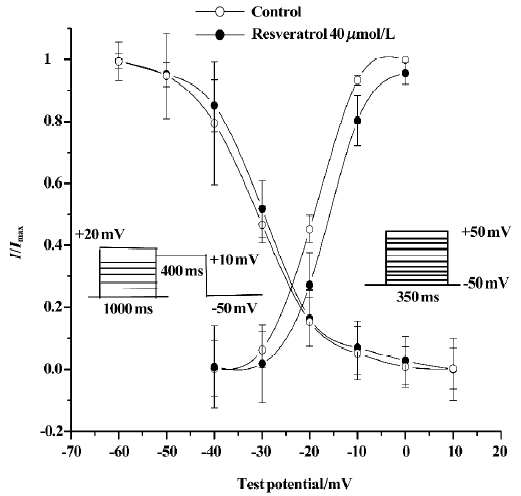
Effects of resveratrol on inactivation of ICa-L Steady-state inactivation of L-type channels was measured by using a double-pulse protocol[14]. Membrane potential was first stepped from -50 mV to +20 mV in 10 mV increments for 1000 ms and then to +10 mV for 400 ms (test pulse) and finally clamped back to the holding potential of -50 mV at a pulse frequency of 0.1 Hz. The peak current elicited by test pulses was normalized against the maximum current and plotted against the conditioning potential. The inactivation curves were also fitted according to the Boltzmann equation. V0.5 is the midpoint voltage of the inactivation functions, and κ is the Boltzmann slope parameter for inactivation. Resveratrol at a concentration of 40 µmol/L shifted V0.5 from -29.9±2.3 mV to -31.1±3.6 mV and κ from 6.3±1.8 mV to 6.8± 3.6 mV (P>0.05; n=6 cells from 5 hearts; Figure 2).
Effect of resveratrol on recovery of ICa-L from inactivation The recovery of ICa-L from inactivation was studied by using a double-pulse protocol consisting of a 300 ms prepulse to +10 mV (P1) followed by a 300 ms test pulse to +10 mV (P2) after a variable P1–P2 coupling interval from 0 to 2500 ms at a holding potential of -60 mV. Double-pulse stimulation was repeated every 6 s. Resveratrol at a concentration of 40 µmol/L markedly shifted the recovery curve of ICa-L to the right and changed the half-recovery time of the Ca2+ channel from 169±19 ms to 197±19 ms (n=6 cells from 6 hearts; P<0.05), indicating slower recovery of ICa-L from inactivation (Figure 3).
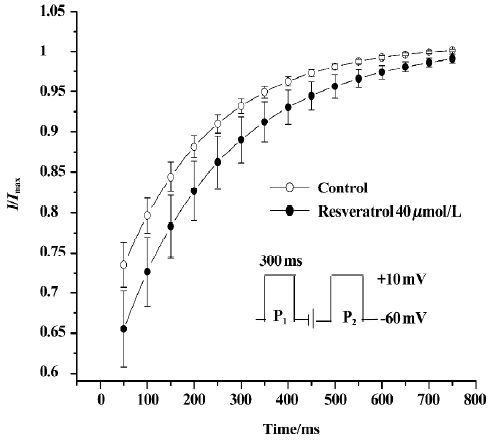
Effects of sodium orthovanadate on resveratrol-induced ICa-L change To further assess the mechanism underlying the inhibitory effect of resveratrol on ICa-L, we observed the effect of sodium orthovanadate (Na3VO4; 1 mmol/L), a potent inhibitor of protein tyrosine phosphatase, on the inhibitory effect of resveratrol. When cells were pretreated with Na3VO4 (1 mmol/L), the resveratrol-induced reduction in ICa-L was significantly attenuated. (n=6 cells from 6 hearts; P<0.01; Figure 4).
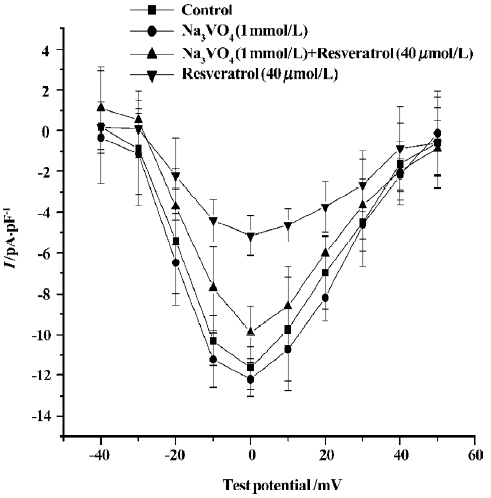
Discussion
In the present study, we found that resveratrol (10, 20, and 40 µmol/L) decreased ICa-L and shifted the I–V curve upward in a concentration-dependent manner. Furthermore, the inhibitory effect of resveratrol disappeared after 15 min washout. This indicates that resveratrol can block L-type calcium channels in rat ventricular myocytes, but the characteristic of I–V curve of ICa-L was not significantly alerted by resveratrol. Resveratrol (40 µmol/L) shifted the steady-state activation curve of ICa-L to the right, suggesting that it can inhibit the steady-state activation of ICa-L; but the inactivation kinetics of ICa-L were not changed by resveratrol (40 µmol/L). In addition, resveratrol (40 µmol/L) markedly shifted the recovery curve of ICa-L to the right, indicating that resveratrol can slow down the recovery of ICa-L from inactiva-tion. These findings indicate that resveratrol decreased ICa-L in rat ventricular myocytes by markedly slowing down the activation of L-type calcium channels and their recovery from inactivation. Therefore, the myocardial electrophysiological effects of resveratrol observed in our previous studies[9,10] might be attributed to its inhibitory effect on ICa-L.
Tyrosine kinase activation is thought to contribute to cell survival, proliferation, and differentiation in many cell types[15]. Furthermore, several lines of evidence show that phosphorylation of tyrosine kinase modulates ion channel activity[16,17]. Some researchers have reported that resveratrol inhibites tyrosine kinase activity, and that many of effects of the resveratrol are mediated by tyrosine kinase[18,19]. Bruder et al reported that resveratrol-induced cellular phenotype was dependent on intracellular calcium and tyrosine kinase activity in bovine pulmonary artery endothelial cells[20]. Furthermore, Conte et al reported that resveratrol could inhibit tyrosine kinase activity in PC12 cells[21]. Sodium orthovanadate, an inhibitor of tyrosine phosphatase, can enhance protein tyrosine phosphorylation[22]. In the present study, we observed the influence of sodium orthovanadate on the effect of resveratrol. We found that pretreatment with sodium orthovanadate markedly antagonized the inhibitory effects of resveratrol on L-type calcium current, suggesting that the tyrosine kinase pathway might be involved in the effects of resveratrol.
In conclusion, we found that resveratrol inhibited ICa-L in rat ventricular myocytes mainly by inhibiting the activation of L-type calcium channels and slowing down the recovery of calcium channels from inactivation. These effects of resveratrol might be mediated via the tyrosine kinase path-way. The inhibitory effects of resveratrol on ICa-L may contribute to its antiarrhythmic actions. This study provides an electrophysiological basis for the use of resveratrol in the treatment of cardiovascular diseases.
References
- Gehm BD, McAndrews JM, Chien PY, Jameson JL. Resveratrol, a polyphenolic compound found in grapes and wine, is an agonist for the estrogen receptor. Proc Natl Acad Sci USA 1997;94:14138-43.
- Lopez-Velez M, Martinez-Martinez F, Del Valle-Ribes C. The study of phenolic compounds as natural antioxidants in wine. Crit Rev Food Sci Nutr 2003;43:233-44.
- Cho DI, Koo NY, Chung WJ, Kim TS, Ryu SY, Im SY, et al. Effects of resveratrol-related hydroxystilbenes on the nitric oxide production in macrophage cells: structural requirements and mechanism of action. Life Sci 2002;71:2071-82.
- Gerhauser C, Klimo K, Heiss E, Neumann I, Gamal-Eldeen A, Knauft J, et al. Mechanism-based in vitro screening of potential cancer chemopreventive agents. Mutat Res 2003;523:163-72.
- Hattori R, Otani H, Maulik N, Das DK. Pharmacological preconditioning with resveratrol: role of nitric oxide. Am J Physiol Heart Circ Physiol 2002;282:H1988-95.
- Zern TL, West KL, Fernandez ML. Grape polyphenols decrease plasma triglycerides and cholesterol accumulation in the aorta of ovariectomized guinea pigs. J Nutr 2003;133:2268-72.
- Jager U, Nguyen-Duong H. Relaxant effect of trans-resveratrol on isolated porcine coronary arteries. Arzneimittelforschung 1999;49:207-11.
- El-Mowafy AM. Resveratrol activates membrane-bound guanylyl cyclase in coronary arterial smooth muscle: a novel signaling mechanism in support of coronary protection. Biochem Biophys Res Commun 2002;291:1218-24.
- Zhao J, Ma HJ, Dong JH, Zhang LP, Liu HL, Wang QS. Electrophysiological effects of resveratrol on guinea pig papillary muscles. Acta Physiol Sin 2004;56:708-12.
- Zhang LP, Ma HJ, Zhao J, Wang QS. Effects of resveratrol on delayed afterdepolarization and triggered activity induced by ouabain in guinea pig papillary muscles. Acta Physiol Sin 2005;57:361-6.
- Dobrydneva Y, Williams RL, Morris GZ, Blackmore PF. Dietary phytoestrogens and their synthetic structural analogues as calcium channel blockers in human platelets. J Cardiovasc Pharmacol 2002;40:399-410.
- Wang Y, Gao J, Mathias RT, Cohen IS, Sun X, Baldo GJ. α-Adrenergic effects on Na+-K+ pump current in guinea pig ventricular myocytes. J Physiol 1998;509:116-28.
- Belles B, Malecot CO, Hescheler J, Trautwein W. “Rundown” of the Ca2+ current during long whole-cell recordings in guinea pig heart cells: role of phosphorylation and intracellular calcium. Pflugers Arch 1988;411:353-60.
- Sun L, Fan JS, Clark JW, Palade PT. A model of the L-type Ca2+ channel in rat ventricular myocytes: ion selectivity and inactivation mechanisms. J Physiol 2000;529:139-58.
- Fantl WJ, Johnson DE, Williams LT. Signalling by receptor tyrosine kinases. Annu Rev Biochem 1993;62:453-81.
- Inoue R, Waniishi Y, Yamada K, Ito Y. A possible role of tyrosine kinase in the regulation of muscarinic receptor-activated cation channels. Biochem Biophys Res Commun 1994;203:1392-7.
- Wijetunge S, Aalkjaer C, Schachter M, Hughes AD. Tyrosine kinase inhibitors block calcium channel currents in vascular smooth muscle cells. Biochem Biophys Res Commun 1992;198:1620-3.
- Jayatilake GS, Jayasuriya H, Lee ES, Koonchanok NM, Geahlen RL, Ashendel CL, et al. Kinase inhibitors from polygonum cuspidatum. J Nat Prod 1993;56:1805-10.
- Palmieri L, Mameli M, Ronca G. Effect of resveratrol and some other natural compounds on tyrosine kinase activity and on cytolysis. Drugs Exp Clin Res 1999;25:79-85.
- Bruder JL, Hsieh T, Lerea KM, Olson SC, Wu JM. Induced cytoskeletal changes in bovine pulmonary artery endothelial cells by resveratrol and the accompanying modified responses to arterial shear stress. BMC Cell Biol 2001;2:1-11.
- Conte A, Pellegrini S, Tagliazucchi D. Effect of resveratrol and catechin on PC12 tyrosine kinase activities and their synergistic protection from beta-amyloid toxicity. Drugs Exp Clin Res 2003;29:243-55.
- Di Salvo J, Semenchuk LA, Lauer J. Vanadate-induced contraction of smooth muscle and enhanced protein tyrosine phos-phorylation. Arch Biochem Biophys 1993;304:386-91.