Inhibitory effect of Ca2+ on in vivo gene transfer by electroporation1
Introduction
Electroporation is a commonly used gene delivery method with relatively high efficiency in vivo[1]. The method has been employed to transfect genes into various tissue types, including skeletal muscle, liver, skin, cornea, vasculature, and tumor tissues[2]. High transfection efficiencies can be achieved relative to other nonviral delivery methods[3]. It is possible that the electroporation delivery process can be further optimized to achieve higher transfection efficiency by varying the electric pulse parameters, the plasmid DNA concentration, the ionic strength, or adding helper molecules such as polymers[4–6]. In particular, it is known that ion composition and concentration in the DNA solution affects the efficiency of electroporation-mediated transfection. Lee et al examined the effect of a wide range of ionic strengths, and found that half saline (71 mmol/L) was best for electroporation in skeletal muscles[7]. In another study, Ca2+ was identified as an important ionic component for electroporation; transgene expressions were shown to be highest when Ca2+ concentrations were in the range 20–100 mmol/L[8].
We reported here our findings using a different experimental protocol, which also suggest the importance of Ca2+ in in vivo electroporation, but the effect we observed was quite the opposite of that observed in the previous study. We found that the presence of Ca2+ in DNA solution significantly inhibited transgene expression after electroporation. By comparing the effects of Ca2+ and various other ions at various concentrations in various experimental settings, we believe that the inhibitory effect of Ca2+ in electro-gene transfer is a real and important phenomenon that has not been discussed before. An in-depth understanding of such a phenomenon may provide some insights into the fundamental mechanism of in vivo electro-gene transfer.
Materials and methods
Plasmid and reagents The luciferase-encoding plasmid under the control of a SV40 promoter and a SV40 enhancer in the pGL3 backbone (pGL3-Control Vector) was bought from Promega (Madison, USA). Plasmid batches were all purified using the Qiagen GIGA kit. CaCl2 and all other reagents were analytical grade and were purchased from Shanghai Chemical Reagent Co and Siji Reagent Co (Shanghai, China).
Animals and animal experiment protocols Female 4- to 6-week-old BALB/c mice were purchased from Fudan University Animal Facility (Shanghai, China). At least 6 animals were included in each experimental group. The animal experiment protocol in the present study was designed in accordance with the regulations of the Chinese Animal Welfare Agency. Before the experiments, mice were anesthetized using 40 mg/mL chloral hydrate at 1% volume relative to body mass. After complete sedation, the gastrocnemius muscle or ventral skin was shaved in each mouse and the mice were given injections of 20 µL 0.1 µg/µL plasmid DNA in various ionic solutions, followed by electroporation treatment using an electric pulse generator made in our laboratory[9]. The electrodes (two silver acupuncture needles, 3 mm apart, each 1 cm long, and 0.3 mm in diameter) were inserted into the muscle or skin covering the site of injection, and 650 ms square wave pulses were delivered at 1 s interval. The field strength was set at 200 V/cm. Transgene expression was usually assayed after 2 d as described below.
Luciferase assay The mice were killed and the gastrocnemius muscles or ventral skin samples were collected for examination. For the luciferase activity assay, tissues were homogenized in 1 mL Promega lysis reagent using a Bead-beater (Cole Pharma, USA). The homogenates were then centrifuged at 2000×g for 6 min and the supernates were assayed using a TD20-20 luminometer (Turner BioSystems, CA), by integration of the light produced during 10 s, starting after the addition of 50 µL of luciferase assay substrate (Promega) to 10 µL supernatant.
Statistical analysis Unless otherwise stated, we tested the significance of the differences between individual groups using the one-tailed Student’s t-test for unpaired values. For statistical comparisons of several groups we used one-way ANOVA. In the figures we report luciferase activity as mean relative light unit (RLU) ±SD.
Results
We examined the effect of different Ca2+ concentrations in the plasmid DNA solution on luciferase expression after electroporation delivery (Figure 1). With only 10 mmol/L of Ca2+ included in the injection medium, transgene expression was reduced to only 2% of the value without Ca2+ (P<0.05, t-test). Further increasing the Ca2+ concentration to 100 mmol/L and 1 mol/L resulted in even lower gene expression. The experiment was repeated a few times using CaCl2 from different sources to avoid any effects of potential chemical impurities, but the inhibitory effect was always large and always reproducible.
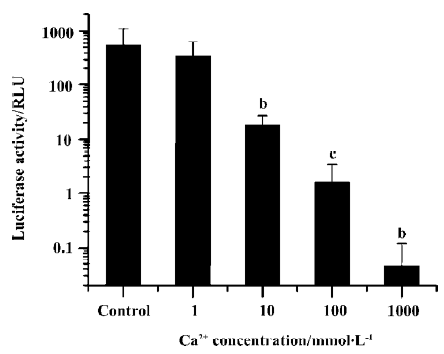
To test whether other ions have similar inhibitory effects, we added Mg2+, Sr2+, Na+, or K+ into the DNA solution instead of Ca2+ at comparable ionic concentrations and assayed for transgene expression after electroporation. Only Ca2+ had a profound inhibitory effect; while all other cations had no effect. In addition, the substitution of the anion Cl- with NO3- in Ca2+-containing solutions did not alter the inhibitory effect (Figure 2).
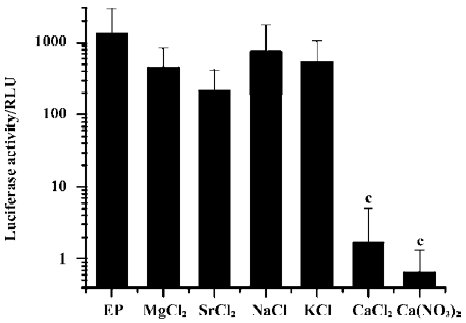
Because the Ca2+ ion plays an important role in the physiological function of muscles, we wondered if the inhibitory effect was unique to muscle tissues. Therefore, we examined the effect of Ca2+ in another tissue type: skin. A mere 5 mmol/L of CaCl2 resulted in an approximately 50-fold reduction in luciferase expression in skin after electroporation (Figure 3).
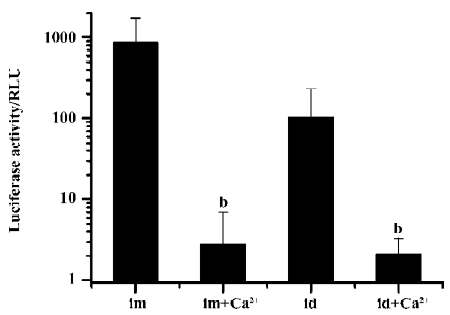
We further examined the effects on transgene expression when the Ca2+-containing buffer was administered separately before or after plasmid DNA injection and electroporation. When the Ca2+-containing buffer was administered by simple needle injection 10 min before the plasmid DNA injection and electroporation, no inhibitory effect was observed. But when the Ca2+-containing buffer was injected and immediately followed by an electroporation treatment, and 10 min later the DNA solution was injected and electroporated, the resulting transgene expression was greatly reduced (Figure 4).
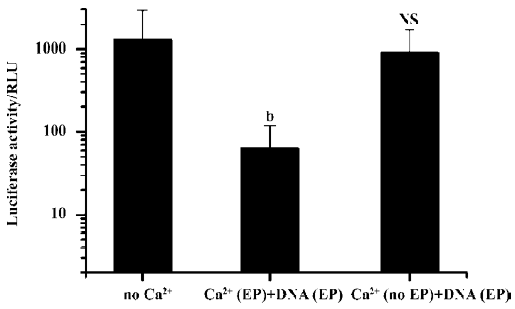
Simple intramuscular needle injection of Ca2+-containing buffer 10 min after plasmid DNA injection and electroporation had no inhibitory effect. But again, if the Ca2+-containing buffers were delivered accompanied by an electroporation treatment, transgene expression was greatly reduced. Even when Ca2+ administration with electroporation was delayed for more than 24 h, the inhibitory effect on transgene expression was still evident (Figure 5).
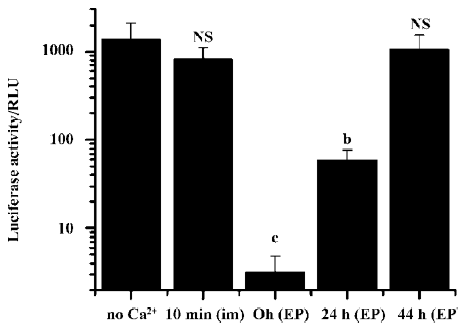
Discussion
Ca2+ is one of the most common divalent cations found in biological fluids. For muscles, the extracellular calcium concentration is estimated to be approximately 2 mmol/L[10]. In the present study, the addition of only 10 mmol/L Ca2+ to the DNA solution (which would be quickly diluted after injection) greatly interfered with transgene delivery and expression, not only in muscles, but also in skin. The higher the Ca2+ concentration was, the lower the transgene expression. Interestingly, other studies on in vitro electroporation for cell transfection concluded that Ca2+ is indispensable in the transfection medium to maintain appropriate ion concentrations[11]. But the results of the present study show that the situation is completely different in vivo.
The mechanism responsible for this inhibitory effect in vivo is unclear. It is possible that calcium might inhibit the activity of luciferase, so to determine whether this was the case, we added 1–5 mol/L of Ca2+ ions into a standard solution of luciferase, but no decrease in luciferase activity was observed (data not shown). Therefore, calcium does not affect the activity of luciferase. Another possibility is that the presence of Ca2+ ions affects the ionic strength as well as the conductivity of the plasmid DNA solution, and therefore alters the movement of DNA molecules in the tissue and into cells. In a recent study by Lee and coworkers, it was suggested that ionic strength was the determining parameter of the effect of Ca2+ on gene transfer. But data from the present study indicated that the inhibitory effect was Ca2+ specific[7]: none of K+, Na+, Mg2+, or Sr2+ at similar ionic strengths had any inhibitory effect. A similar argument could also be used to rule out the possibility that the divalent Ca2+ interacted with plasmid DNA via a charge interaction, which altered the stability and physical properties of DNA molecules. In addition, by injecting Ca2+ separately from the DNA solution, we also minimized the interaction between Ca2+ ions and plasmid DNA, but the inhibitory effect was still evident.
The data gathered during the separate injection experiment were quite striking. We found that Ca2+-containing buffers could be delivered as late as 24 h after delivery of the plasmid to cause the inhibitory effect. Another important observation is that the inhibitory effect was only evident when Ca2+ was also delivered by electroporation, suggesting that Ca2+ possibly needs to enter the cells in order to exert its inhibitory effect. When delivered by simple needle injection, Ca2+ ions would be mostly present in the extracellular space, so the inhibitory effect would be limited.
The electroporation process would cause cell membrane disruption, which would allow Ca2+ influx into the cells to result in damage to the muscle cells[12]. During our experi-ments, we inspected the damage caused by electro-poration with or without Ca2+. There was significant damage in the muscle fibers caused by electroporation. However, there was no noticeable difference in the amount of damage with or without the addition of Ca2+. However, it is still possible that the Ca2+ caused damage that was not detected by us.
Another possibility is that Ca2+ influx exerted an effect on cell membrane stability. A recent report suggested that the Ca2+ influx may initiate a membrane resealing mechanism[13]. In that study, cell behaviors were studied after ultrasound treatment, which is supposed to cause pores in the cell membrane. It was found that resealing of the cell membrane required extracellular Ca2+ to enter cells through the pores. So in our experiments, it is possible that the administration of different concentrations of Ca2+ ions effectively resulted in changes in cell membrane permeability to DNA molecules. The pores in the cell membrane caused by electro-pulses resealed quickly after Ca2+ influx to limit plasmid DNA entrance into the cells. Such a theory would also explain the importance of extracellular Ca2+ in in vitro electroporation mediums, because in vitro electroporation conditions are harsher and are thought to result in more cell deaths. However, the exact mechanism for Ca2+ influx-induced cell membrane pore resealing is not clear, and requires further investigation.
In summary, we hope that the present study will attract more attention on the complexity of optimizing in vivo gene transfection by electroporation. Further studies to investigate the details of these effects will eventually build up a clearer picture of the underlying mechanism of this promising technology.
References
- McMahon JM, Wells DJ. Electroporation for gene transfer to skeletal muscles: current status. BioDrugs 2004;18:155-65.
- Li S, Benninger M. Applications of muscle electroporation gene therapy. Curr Gene Ther 2002;2:101-5.
- Gardlik R, Palffy R, Hodosy J, Lukacs J, Turna J, Celec P. Vectors and delivery systems in gene therapy. Med Sci Monit 2005;11:110-21.
- Gehl J, Mir LM. Determination of optimal parameters for in vivo gene transfer by electroporation, using a rapid in vivo test for cell permeabilization. Biochem Biophys Res Commun 1999;261:377-80.
- Mir LM, Bureau MF, Gehl J, Rangara R, Rouy D, Caillaud JM, et al. High-efficiency gene transfer into skeletal muscle mediated by electric pulses. Proc Natl Acad Sci USA 1999;96:4262-7.
- Lucas ML, Jaroszeski MJ, Gilbert R, Heller R. In vivo electroporation using an exponentially enhanced pulse: a new waveform. DNA Cell Biol 2001;20:183-8.
- Lee MJ, Cho SS, Jang HS, Lim YS, You JR, Park J, et al. Optimal salt concentration of vehicle for plasmid DNA enhances gene transfer mediated by electroporation. Exp Mol Med 2002;34:265-72.
- Suzuki T, Tsunekawa J, Murai A, Muramatsu T. Effect of CaCl2 concentration on the rate of foreign gene transfer and expression by in vivo electroporation in the mouse ovary. Int J Mol Med 2003;12:365-8.
- Xu L, Zhao Y, Zhang Q, Li Y, Xu Y. Regulation of transgene expression in muscles by ultrasound-mediated hyperthermia. Gene Ther 2004;11:894-900.
- Church PJ, Stanley EF. Single L-type calcium channel conductance with physiological levels of calcium in chick ciliary ganglion neurons. J Physiol 1996;496:59-68.
- Neumann E, Kakorin S, Tsoneva I, Nikolova B, Tomov T. Calcium-mediated DNA adsorption to yeast cells and kinetics of cell transformation by electroporation. Biophys J 1996;71:868-77.
- Gissel H, Clausen T. Ca2+ uptake and cellular integrity in rat EDL muscle exposed to electrostimulation, electroporation, or A23187. Am J Physiol Regul Integr Comp Physiol 2003;285:132-42.
- Deng CX, Sieling F, Pan H, Cui J. Ultrasound-induced cell membrane porosity. Ultrasound Med Biol 2004;30:519-26.