Hydrogen sulfide facilitates carotid sinus baroreflex in anesthetized rats
Introduction
Hydrogen sulfide (H2S) is a toxic gas that is found in contaminated environments. Many studies have shown that H2S affected the nervous system and vascular and intestinal smooth muscle of mammals[1–6]. Although toxic at high doses, there was increasing evidence to suggest that H2S has important physiological functions in the mammalian cardiovascular system[4]. The production of H2S from L-cysteine can be catalyzed by two pyridoxal-5'-phosphate-dependent enzymes, cystathionine β-synthase (CBS) and cystathionine α-lyase (CSE), in mammalian tissue[7–9]. The expression of these two enzymes is tissue-type specific[4]. H2S is directly produced in arterial and venous tissue by CSE[10,11]. DL-propargylglycine (PPG) is a specific inhibitor of CSE, which can suppress endogenous H2S production[12].
H2S has been proposed to be an endogenous gaseous transmitter similar to 2 other vasoactive gases, NO (nitric oxide) and CO (carbon monoxide). The vascular effect of H2S is exerted by direct activation of KATP channels and hyperpolarization of the membrane potential of vascular smooth muscle cells (VSMCs)[10]. It has been found that the vascular effect of H2S was partially mediated by a functional endothelium, and its action was dependent on extracellular calcium entry, but is independent of activation of the cGMP pathway, unlike NO and CO[13]. Recently, H2S has been found to play a negative inotropic role in the heart[14].
The baroreflex is the major method of blood pressure modulation. Whether H2S affects the carotid sinus baroreflex (CSB) remains to be clarified. The aim of our study was to observe the action of exogenous H2S, derived from sodium hydrosulfide (NaHS), and endogenous H2S on the isolated CSB and to elucidate the mechanism involved.
Materials and methods
Drugs NaHS, Bay K8644 and PPG were purchased from Sigma. NaHS was used as a donor of H2S. Glibenclamide (a KATP channel blocker) was purchased from the Tianjin Institute of Medical and Pharmaceutical Industry. Glibenclamide was initially dissolved in dimethylsulfoxide (100 µmol/L). The final concentration of dimethylsulfoxide in the K-H solution was 0.01% (v/v). No change was observed in the CSB during perfusion with the final concentration of dimethylsul-foxide. Bay K8644 (an L-type calcium channel agonist) was dissolved in 99% ethyl alcohol. No changes in the CSB were observed during perfusion with ethyl alcohol (1:2000). PPG (an inhibitor of CSE) was dissolved in distilled water.
General surgical procedure Sprague-Dawley rats (male, 320±20 g), which were obtained from the Experimental Animal Center of Hebei Province, were anesthetized with 25% urethane (1.0 g/kg, ip). In each rat, the trachea was cannulated for ventilation, and the right femoral artery was cannulated for recording blood pressure (BP) with a transducer (MPU-0.5A; Nihon Kohden). Body temperature was maintained at 37–38 °C throughout the experiment.
Perfusion of left isolated carotid sinus The perfusion of isolated carotid sinus area was carried out using a previously reported method with modifications[15]. Carotid sinus areas were fully exposed by turning the trachea and esophagus in the rostral direction. The sternohyoideus muscles and superior laryngeal nerves were cut, then the bilateral aortic nerves, right carotid sinus nerve, cervical sympathetic nerves and recurrent laryngeal nerves were all sectioned. The common, external and internal carotid arteries and smaller arteries originating from these vessels were exposed and ligated, while carefully leaving the left carotid sinus nerve undisturbed. Ligation of the occipital artery at its origin from the external carotid artery excluded chemoreceptors from the isolated carotid sinus, thereby preventing chemoreceptor activation secondary to decrease in carotid sinus pres-sure. Plastic catheters were inserted anterograde into the left common carotid artery (inlet tube) and retrograde into the external carotid artery (outlet tube). The carotid sinus was then perfused with warm (37 °C) oxygenated modified Krebs-Henseleit (K-H) solution (in mmol/L; NaCl 118.0, KCl 4.7, CaCl2 2.5, MgSO4 1.6, KH2PO4 1.2, NaHCO3 25, glucose 5.6, pH 7.35–7.45) bubbled with 95% O2 and 5% CO2. The intrasinus pressure (ISP) was monitored by using a pressure transducer (MPU-0.5A; Nihon Kohden) connected to the inlet tube. The ISP was controlled by using a peristaltic pump.
After perfusion of the left carotid sinus, the ISP was kept at 100 mmHg for 20 min and was then lowered to 0 mmHg rapidly. From this point, the ISP was elevated to 250 mmHg via a pulsatile ramp by regulating the speed of the peristaltic pump, which was automatically controlled by a program designed by our laboratory[16]. It took 0.5 min for the ISP to be increased from 0 to 250 mmHg. The ISP and BP were simultaneously recorded on a polygraph (RM-6240; Chengdu Instrument Factory). This process was repeated at an interval of 5 min to check the stability of the baroreflex. The reproducibility of the experimental set-up was confirmed by the recurrent drop of BP in response to the increase in ISP.
Experimental protocols By perfusing the left carotid sinus with K-H solution and elevating the ISP, a functional curve for the ISP-BP relationship was constructed, and the functional parameters of baroreflex, such as threshold pressure (TP), saturation pressure (SP), equilibrium pressure (EP), peak slope (PS), reflex decrease of BP (RD), and operating range (OR) were determined. TP was the ISP at which BP began to decrease in response to the increase of the ISP. SP was the ISP at which BP just showed no further reflex decreases with an increase in the ISP. OR was calculated as SP minus TP.
Before administration of the drugs, the K-H solution was used as a control. Four experimental treatments were used. (1) To test the effect of NaHS on carotid baroreflex (n=18), the ISP was fixed at 100 mmHg for 20 min with K-H solution as a control, and the baroreflex parameters were measured. Then K-H solution containing NaHS (25, 50, or 100 µmol/L) was used to perfuse the isolated carotid sinus for 50 min, then the parameters were measured again. Finally, the carotid sinus was perfused with K-H solution to wash out the NaHS. (2) To test the effect of glibenclamide (Gli; 20 µmol/L) on the actions of NaHS (n=6), baroreflex parameters were examined following the application of NaHS before and after pretreatment with Gli for 20 min. (3) To test the effect of Bay K8644 (500 nmol/L) on the actions of NaHS (n=6), baroreflex parameters were examined following the application of NaHS before and after pretreatment with Bay K8644 for 15 min. (4) To test the effect of PPG (200 µmol/L) on the carotid baroreflex (n=6), after the baroreflex parameters of the control were recorded, PPG was added into a K-H solution and used to perfuse the isolated carotid sinus, then washed out. Finally, the carotid sinus was perfused with K-H solution containing NaHS (50 µmol/L) to check the carotid baroreflex activity.
Data analysis All data are expressed as mean±SD. The differences between groups of means were assessed by one-way ANOVA and further analyzed using the Student-Newman-Keuls test. P<0.05 was considered statistically significant.
Results
Effects of NaHS on carotid sinus baroreflex By perfusing the left carotid sinus with K-H solution and elevating the ISP from 0 to 250 mmHg, BP was reflex decreased. NaHS induced obvious changes in baroreflex parameters, which appeared approximately 30 min after perfusing the isolated carotid sinus with K-H solution containing NaHS, and disappeared 30–60 min after washout. Compared with the control group, NaHS increased RD and PS in a concentration-dependent manner, and decreased TP and EP, shifting the functional curve of the baroreflex downward and to the left (Table 1, Figure 1). The functional curve was fit using Origin 6.0 procedures. The effects described indicate that exogenous NaHS exerts a facilitatory effect on the carotid baroreflex (Figure 2).
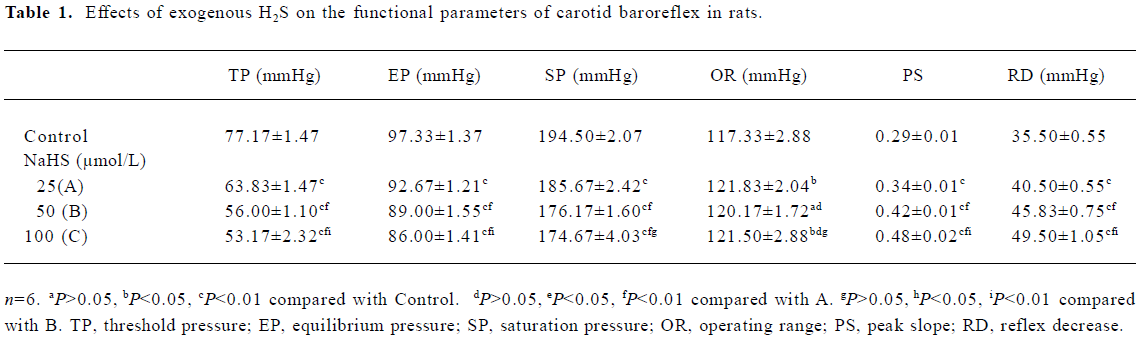
Full table
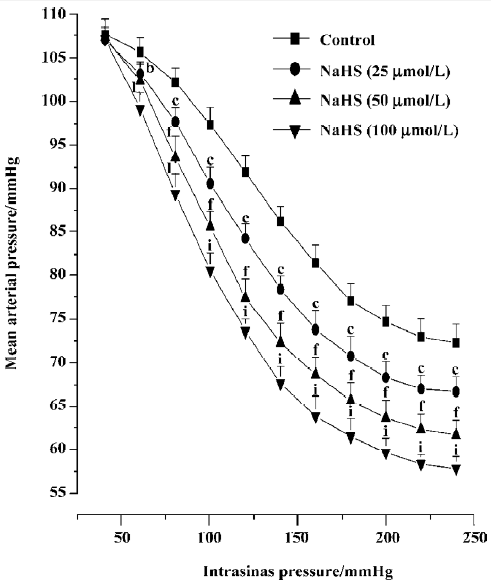
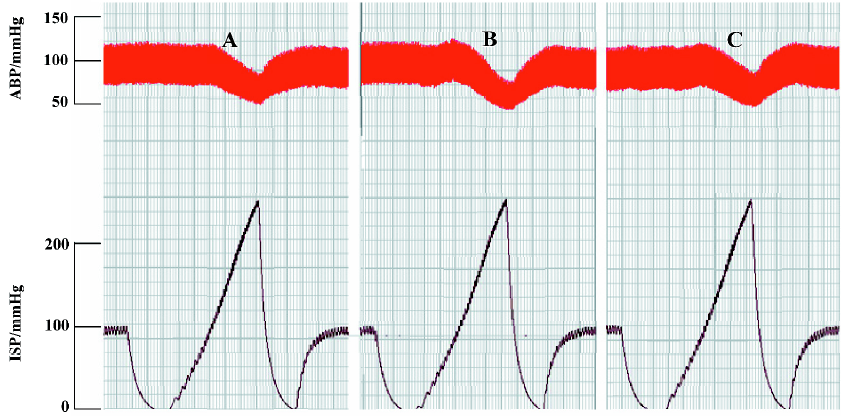
Effects of Gli and Bay K8644 on the actions of NaHS Neither Gli (20 µmol/L) nor Bay K8644 (500 nmol/L) induced any changes in the functional parameters of the baroreflex, but both completely blocked the effects of NaHS (Table 2).
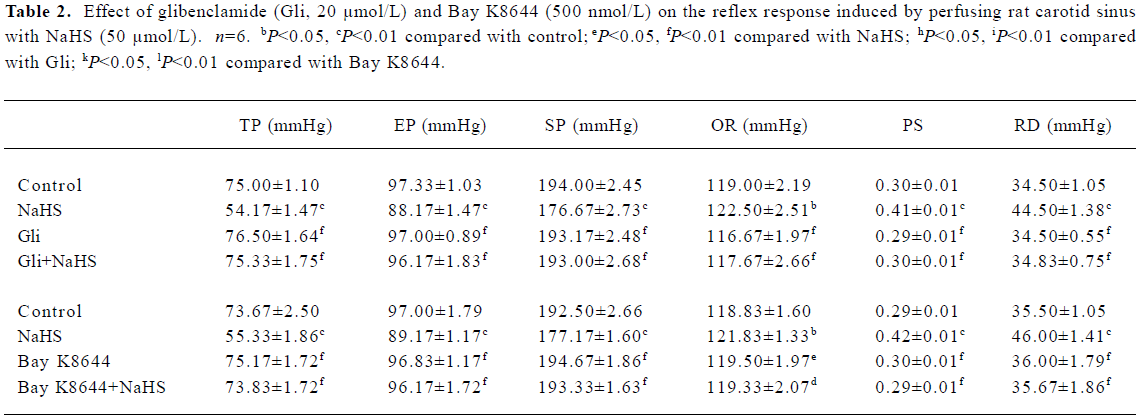
Full table
Effect of PPG on carotid sinus baroreflex PPG inhibited the CSB in male rats and shifted the functional curve of the baroreflex upward and to the right (Table 3).
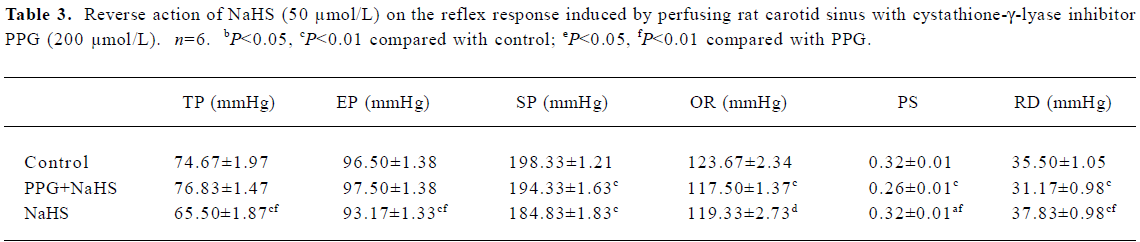
Full table
Discussion
The present study showed that H2S could facilitate the CSB in a dose-dependent manner. By perfusing the left isolated carotid sinuses of rats with exogenous H2S derived from NaHS, the functional curve of the CSB was shifted downward and to the left, with increases in PS and RD and decreases in TP, indicating the facilitatory action of H2S on the CSB.
It has been shown that H2S has cardiovascular effects, and that H2S can relax isolated aortic tissue in vitro in a KATP channel-dependent manner. Furthermore, in isolated VSMCs, H2S can directly increase KATP channel currents and hyperpolarize membrane[10]. So H2S is considered to be an important endogenous vasoactive factor and the first identified gaseous opener of KATP channels in vascular smooth muscle cells. It is known that KATP channels exist in vascular smooth muscle[17]. A previous study from our laboratory reported that the opener of KATP channels can facilitate the carotid baroreflex[18]. On the basis of these data, we added glibenclamide (20 µmol/L), a KATP channel blocker, to the perfusate and found that it completely eliminated the effect of H2S on the carotid baroreflex. Such results suggest that the opening of the KATP channel is involved in the action of H2S on the CSB.
Brayden recently reported that KATP channel opening could hyperpolarize smooth muscle, which led to closure of the voltage-dependent Ca2+ channels, thereby causing a reduction in intracellular Ca2+ and vasodilation[19]. It is also known that distention of the carotid sinus can activate mechanosensitive ion channels, which would enhance the activity of the baroreceptors[20]. In the present study, pretreatment with L-type calcium channel agonist Bay K8644 completely abolished the facilitating effect of H2S on CSB. Based on the above observations, it can be concluded that H2S facilitates CSB through opening KATP channels and further closing calcium channels in VSMCs.
The results so far discussed only relate to the actions of exogenous H2S. In order to determine the function of endogenous H2S, PPG (an inhibitor of CSE) was used in our experiment. Zhao reported that PPG might be a membrane-permeable drug, and that it had the potential to be used to study the physiological function of endogenously produced H2S[12]. In the present study, PPG shifted the functional curve of CSB upward and to the right, with decreases in PS and RD, and increases in TP. These results indicate that endogenous H2S may have an activating role with respect to CSB activity in vivo.
It is known that arterial baroreceptors play an important role in the short-term modulation of CSB activity. Facilitating the CSB would tend to decrease arterial blood pressure, which could antagonize the hypertension caused by other stimulators. Some diseases, such as hypoxic pulmonary hypertension[21], result from a deficiency in endogenous H2S production, so the modulation activity of the CSB is suppressed and the arterial blood pressure will increase. Deficits in the H2S/CSE system are responsible for the development of spontaneous hypertension accompanying aorta remodeling[22,23]. Exogenous supply of an H2S donor may exert a protective effect in the pathogenesis of hypertension.
In summary, H2S facilitated the carotid baroreflex, which may result from the opening of KATP channels and further closing of the calcium channels. It is possible that H2S activates the carotid baroreflex in physiological conditions.
References
- Beauchamp RJ, Bus JS, Popp JA, Boveiko CJ, Andielkovich DA. A critical review of the literature on hydrogen sulfide toxicity. CRC Crit Rev Toxicol 1984;13:25-97.
- Guidotti TL. Hydrogen sulfide. Occup Med 1996;46:367-71.
- Warenycia MW, Goodwin LR, Benishin CG, Reiffenstein RJ, Francom DM, Taylor JD, et al. Acute hydrogen sulfide poisoning: demonstration of selective uptake of sulfide by the brain stem by measurement of brain sulfide levels. Biochem Pharmacol 1989;38:973-81.
- Wang R. Two’s company, three’s a crowd: can H2S be the third endogenous gaseous transmitter? FASEB J 2000;16:1792-8.
- Abe K, Kimura H. The possible role of hydrogen sulfide as an endogenous neuromodulator. J Neurosci 1996;16:1066-71.
- Kimura M. Hydrogen sulfide as a neuromodulator. Mol Neurobiol 2002;26:13-9.
- Bukovska G, Kery V, Krous JP. Expression of human cystathionine beta-synthase in Escherichia coli purification and characteriza-tion. Protein Expr Purif 1994;5:442-8.
- Stipanuk MH, Beck PW. Characterization of the enzymic capacity for cysteine desulphydration in liver and kidney of the rat. Biochem J 1982;206:267-77.
- Swaroop M, Bradley K, Ohura T, Tahara T, Roper MD, Rosenberg LE, et al. Rat cystathionine β-synthase. Gene organization and alternative splicing. J Biol Chem 1995;267:11455-61.
- Zhao WM, Zhang J, Lu YJ, Wang R. The vasorelaxant effect of H2S as a novel endogenous gaseous KATP channel opener. EMBO J 2001;20:6008-16.
- Hosoki R, Matsuki N. kimura H. The possible role of hydrogen sulfide as an endogenous smooth muscle relaxant in synergy with nitric oxide. Biochem Biophys Res Commun 1997;273:527-31.
- Zhao WM, Joseph FN, Wang R. Modulation of endogenous production of H2S in rat tissues. Can J Physiol Pharmacol 2003;81:848-53.
- Zhao WM, Wang R. H. 2S-induced vasorelaxation and underlying cellular and molecular mechanisms. Am J Physiol 2002;283:H474-80.
- Geng B, Yang JH, Qi YF, Zhao J, Pang YZ, Du JB, et al. H2S generated by heart in rat and its effects on cardiac function. Biochem Biophys Res Commun 2004;313:362-8.
- Zhao G, He RR. The facilitating effect of atrial natriuretic peptide on the carotid sinus baroreflex function. Chin J Physiol Sci 1993;9:68-75.
- Yi XL, Fan ZZ, Ho SY. An automatic system controlled by computer for carotid sinus perfusion. Chin J Appl Physiol 1993;9:156-9.
- Standen NB, Quayle JM, Davies NW, Brayden JE, Huang Y, Nelson MT. Hyperpolarizing vasodilators activate ATP-sensitive K+ channels in arterial smooth muscle. Science 1989;245:177-80.
- Yin T, Chen S, He RR. K. ATP channel opener facilitates carotid sinus baroreflex in anaesthetized rats. Acta Physiol Sin 2000;52:170-4.
- Brayden JE. Functional roles of KATP channels in vascular smooth muscle. Clin Exp Pharmacol Physiol 2002;29:312-6.
- Chapleau MW, Li Z, Meyrelles SS, Abboud FM. Mechanisms determining sensitivity of baroreceptor afferents in health and disease. Ann NY Acad Sci 2001;940:1-19.
- Zhang CY, Du JB, Bu DF, Yan H, Tang XY, Jan CS. The regulatory effect of hydrogen sulfide on hypoxic pulmonary hypertension in rats. Biochem Biophys Res Commun 2003;302:810-6.
- Yan H, Du JB, Tang CS. The possible role of hydrogen sulfide on the pathogenesis of spontaneous hypertension in rats. Biochem Biophys Res Commun 2004;313:22-7.
- Zhong GZ, Chen FR, Cheng YQ, Tang CS, Du JB. The role of hydrogen sulfide generation in the pathogenesis of hypertension in rats induced by inhibition of nitric oxide synthase. J Hypertension 2003;21:1879-85.