Electrophysiological effect of fluoxetine on Xenopus oocytes heterologously expressing human serotonin transporter1
Introduction
Neurotransmitter transporters play a very important role in terminating synaptic transmission. After the neurotransmitters are released into the synaptic cleft and activate the receptors, there are 3 ways to eliminate the function of neurotransmitters: (1) some neurotransmitters, such as acetylcholine (ACh), are decomposed by enzymes; (2) some are carried away by the blood; and (3) others, such as monoamine and amino acid transmitters, undergo reuptake by the transporters located in the plasma membrane of neurons[1]. Transporters are very interesting for 2 reasons: they succeed at an extraordinarily difficult job (pumping transmitter molecules across a membrane), and they are the molecular site at which many important psychoactive drugs act[2]. Serotonin (5-hydroxytryptamine; 5-HT) is a monoamine transmitter, and it is mostly removed from the synaptic cleft by reuptake into the presynaptic membrane via the serotonin transporters (SERT). A low level of serotonin in the brain is one of the causes of depression. The most popular antidepressant drugs are: (1) tricyclic compounds, such as imipramine, which block the reuptake of both norepinephrine and serotonin; (2) monoamine oxidase (MAO) inhibitors, such as phenelzine, which reduce the enzymatic degradation of serotonin and norepinephrine; (3) selective serotonin reuptake inhibitors (SSRI), which affect the presynaptic terminals.
Fluoxetine, an SSRI, acts only on the serotonin terminals[3] rather than on other monoamine transporters[4]. The clinical dosage of fluoxetine is much smaller than that of other antidepressant drugs, such as desipramine[5]. Fluoxetine elevates the levels of 5-HT in the brain, but its therapeutic actions take weeks to develop[6]. It is unknown why fluoxetine is so effective in blocking SERT and why it has a longer-lasting blocking effect than other antidepressant drugs, such as desipramine and paroxetine. Although many of the clinical pharmacological effects of fluoxetine have been well characterized in different species and by using different techni-ques[5,7–9], little information is available regarding its electrophysiological properties. It is still unclear how fluoxetine reverses the 5-HT-induced currents.
By using human serotonin transporters (hSERT) heterologously expressed in Xenopus oocytes, and the 2-electrode voltage clamp technique, we studied the dynamic changes of hSERT in the process of transporting 5-HT under the influence of fluoxetine.
Materials and methods
Oocytes and cRNA Stage V and VI oocytes were harvested from adult Xenopus laevis females and prepared following the method of Machacak and Hartzell[10]. Human serotonin transporter (hSERT or 5-HTT) cRNA was transcribed from cDNA, which was cloned using a pOTV vector. The hSERT cDNA was donated by Vanderbilt University, Nashville,TN, USA. Stage V and VI defolliculated oocytes were injected with 52 nL (1 ng/nL) hSERT cRNA and then incubated at 19 °C in Ringer’s solution (pH 7.6) supplemented with 1% penicillin-streptomycin stock and 5% horse serum. Oocytes were used 7–10 d after isolation and injection[11].
Electrophysiology Oocytes were voltage-clamped with 2 microelectrodes using an Axonpatch 200B amplifier (Axon Instruments, Foster city, USA) band-limited at 10 kHz. Microelectrodes were pulled using a programmable puller (Sutter Instruments, USA) such that their resistances were 1–2 MΩ when filled with 3 mol/L KCl solution and dipped into the bath solution. The bath solution was Na Ringer’s solution, which comprised (in mmol/L): 96 NaCl, 2 KCl, 5 MgCl2, 5 N-2-hydroxyethylpiperazine-N'-2-ethanesulfonic acid (HEPES) and 0.6 CaCl2 (pH 7.6). A gravity pump operating at 1 mL/min was used to change the bath solution. Current records were stored on a computer and analyzed with the Origin 6 software package (Microcal Software). The holding potential was -50 mV, unless otherwise noted in the text or figure legends. All the data were obtained at room temperature (21–23 °C).
In the experiments, the oocytes were first perfused with Ringer’s solution, then perfused with Ringer’s solution containing 5-HT (10 µmol/L) to induce an inward current (5-HT-induced hSERT-current). Different concentrations of fluoxetine were added into the Ringer’s solution containing 5-HT to reverse the 5-HT-induced currents. Finally, the perfusion solution was changed back to Ringer’s solution. A fitted exponential function was used to obtain the time constants of the hSERT-currents.
Results
Reversal of 5-HT-induced hSERT-current by fluoxetine 5-HT at a concentration of 10 µmol/L was used to induce an inward current (Figure 1A). 5-HT-induced hSERT current can be reversed by fluoxetine (Figure 1B). The reversal time (τ3) of fluoxetine was much longer than the time taken for the 5-HT-induced hSERT current to reach its peak value (τ1) or the recovery time for the 5-HT-induced current to be washed out by Ringer’s solution (τ2, Figure 1). The dissociation time constant of 5-HT-induced hSERT current and the reversal time constant of fluoxetine were 8.74 s and 23.56 s, respectively (Figure 1A, 1B). It seems that fluoxetine requires more time to reverse the function of hSERT than that required for 5-HT to combine with or dissociate from hSERT. If the oocytes were perfused with fluoxetine alone, there were no obvious inward or outward currents observed.
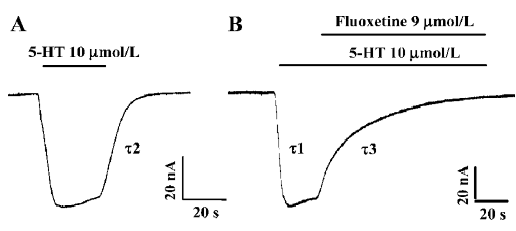
Concentration-dependent reversal of 5-HT-induced hSERT-current The mean percentage reversal effect of fluoxetine at different concentrations on hSERT-current were calculated. An exponential function was used to fit the curve. The fitted curve revealed the dose-dependent relationship between fluoxetine concentration and the percentage reversal of hSERT-current. Based on the fitted curve, RC50 (50% reversal concentration) was 3.12 µmol/L (Table 1).
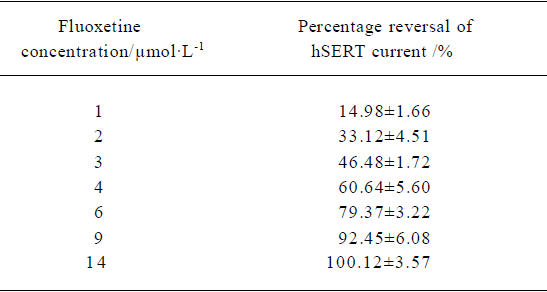
Full table
Reversal time constant versus the concentration of fluoxetine By using different concentrations of fluoxetine, we found that the time constants for reversing hSERT currents to steady state were dose-dependent (Figure 2). As the concentration of fluoxetine increased, the reversal time course became shorter. The time course shown in Figure 2A, using a concentration of 1 µmol/L, is much longer than those for concentrations of 3 and 6 µmol/L, as shown in Figure 2B and 2C, respectively. Table 2 shows the mean values and the standard errors of the time constants when the oocytes were treated with different concentrations of fluoxetine.
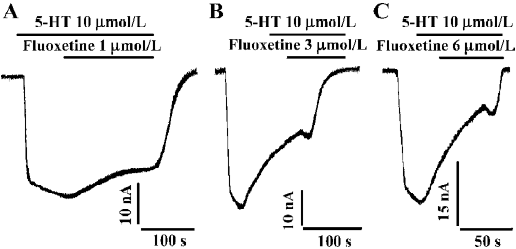
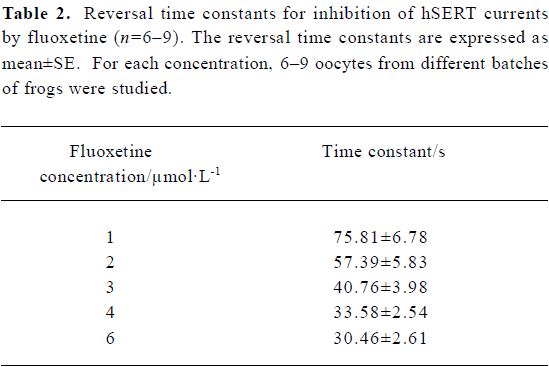
Full table
Long-lasting effect of fluoxetine on the hSERT When the 5-HT-induced hSERT current was first reversed with fluoxetine, then washed away with Ringer’s to the control level, and later the same perfusion protocol was repeated, we found that on the second perfusion with the same concentration of 5-HT, the time constant for 5-HT-induced current became much greater than that for the first perfusion (Figure 3). Relative to the first perfusion, the time constant for 5-HT-induced hSERT current increased from 4.72 s to 83.68 s (Figure 3). But for both perfusions, there was no difference in the reversal effect of fluoxetine on the hSERT current (Figures 3 and 4): the reversal time constants were 25.32 s and 26.97 s, respectively. Our results also indicated that the long-lasting effect of fluoxetine was dose-dependent (Table 3).
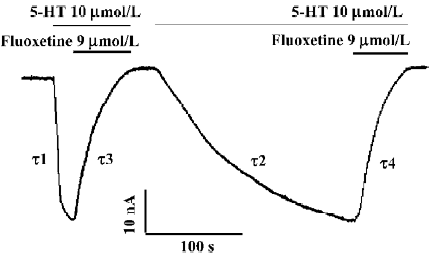
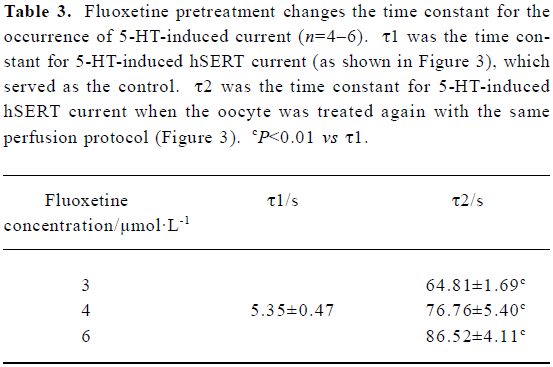
Full table
Discussion
Before performing the experiment with fluoxetine, we first had to prove that the 5-HT-induced current was hSERT current[11]. 5-HT is co-transported with Na+ and Cl– ions. If the holding potentials in the voltage clamping are changed, with a constant concentration of perfused 5-HT, the amplitude of the current should change accordingly. We observed that the more negative the holding potential used, the larger the inward current, which demonstrated that this was a voltage-dependent current. Furthermore, the 5-HT-induced current could be totally blocked by desipramine, a specific blocker of the monoamine transporter (data not shown), which also confirmed that this current was heteroexpressed hSERT-current.
After the 5-HT-induced current reached a stable state, fluoxetine was added into the perfusion solution. The 5-HT-induced current then became smaller and smaller (Figure 1B), and finally the current trace returned to zero, which indicated that the 5-HT-induced current was almost completely blocked by fluoxetine at a concentration of 9 µmol/L.
We found that the blocking effect of fluoxetine was not as fast as we expected. The blocking time (τ3) was much longer than the time needed for 5-HT-induced current to reach its maximum value (τ1; Figure 1), because the time constant for the former was 23.56 s and for the later it was 3.88 s.
The 5-HT current could be dose-dependently reversed by fluoxetine; the RC50 was approximately 3.12 µmol/L. The reversal time constant varied with different concentrations of fluoxetine (Table 2). The higher the concentration of fluoxetine, the faster the reversal process.
We observed that the reversal effects of fluoxetine are characterized by 3 main features:
First, Compared with the time course from washing out the 5-HT with control Ringer’s solution (Figure 1A, 1B), the reversal time constant of fluoxetine was much longer than those produced by Ringer’s solution, which suggests that fluoxetine is competing with 5-HT for the binding sites in the transporter, resulting in a decreased current. However, the molecular binding site of fluoxetine needs further investiga-tion.
Second, when the hSERT-expressing oocyte was repeatedly perfused with fluoxetine and then washed out with Ringer’s solution, the time constant for the occurrence of 5-HT current during the second or third perfusion became much greater than that for the first perfusion (τ2>τ1; Figure 3) and even greater than the reversal time constant of fluoxetine (τ2>τ4). It has been reported that fluoxetine has high affinity to hSERT (Ki=33 nmol/L)[13] . This could explain why fluoxetine had a long-lasting effect on hSERT (τ2>τ1; Figure 3) in our experiments.
Third, all reversal processes, no matter what concentration of fluoxetine was used, could be fitted with a single exponential function. The exponential function B=B0+B1e-k1t+B2e-k2t +.. allowed us to calculate the combination or dissociation rate constants of receptor-ligand complexes and speculate regarding the dynamic mechanisms involved[12]. The time course of the current reversals reflected the combination of fluoxetine with hSERT. The single exponential function fitting suggests that fluoxetine reverses the 5-HT-induced-current by competing with the binding of 5-HT to hSERT. Other mechanisms of antidepressant therapy[6], such as regulating the density of hSERT, activating neurotrophins or glucocorticoids in the cells, and modulating the intracellular signal pathways, may not be involved in our experiments. If they had been, there might have been more than one time constant observed.
In clinical practice, fluoxetine is used to treat patients who have reduced amounts of 5-HT in the brain. Because fluoxetine can suppress the function of 5-HT transporters, it would leave more 5-HT in the synaptic clefts. Its long-lasting effect on hSERT might explain why patients take much more desipramine (up to 200 mg/d) than fluoxetine (20 mg/d) in clinical treatments[5]. The lower fluoxetine dosage used could reduce the occurrence of side-effects such as fever, headache, naupathia, diarrhea, and dyspnea.
References
- Lester HA, Mager S, Quick MW, Corey JL. Permeation properties of neurotransmitter transporters. Annu Rev Pharmacol Toxicol 1994;34:219-49.
- Bear MF, Connors BW, Paradiso MA. Neuroscience: Exploring the brain. 2nd ed. New York: Lippincott, Williams and Wilkins; 2001.
- Blier P, De Montigny C. Current advances in the treatment of depression. Trends Pharmacol Sci 1994;15:220-6.
- Cusack B, Nelson A, Richelson E. Binding of antidepressants to human brain receptors: focus on never generation compounds. Psychopharmacology (Berlin) 1994;114:559-65.
- Walker Z, Walker RW, Robertson MM, Stansfeld S. Antidepressant treatment of chronic tension-type headache: a comparison between fluoxetine and desipramine. Headache 1998;38:523-8.
- Patrick S, Fritz AH. New insights into the mechanisms of antidepressant therapy. Pharmacol Ther 2004;102:47-60.
- Rudd ML, Nicolas AN, Brown BL, Fischer-Stenger K, Stewart JK. Peritoneal macrophages express the serotonin transporter. J Neuroimmunol 2005;159:113-8.
- Meyer JH, Wilson AA, Sagrati S, Hussey D, Carella A, Potter WZ, et al. Serotonin transporter occupancy of five selective serotonin re-uptake inhibitors at different doses: an [11C] DASB positron emission tomography study. Am J Psychiatry 2004;161:826-35.
- Hirano K, Kimura R, Sugimoto Y, Yamada J, Uchida S, Kato Y, et al. Relationship between brain serotonin transporter binding, plasma concentration and behavioural effect of selective serotonin reuptake inhibitors. Br J Pharmacol 2005;144:695-702.
- Machaca K, Hartzell HC. Asymmetrical distribution of Ca activated Cl channel in Xenopus Oocytes. Biophys J 1998;74:1286-95.
- Wang YY, Jin Z, Li CZ, Liu YM. Establishment of heterologous expression model of hSERT in Xenopus laevis oocytes. Chin J Appl Physiol 2005;21:30-4.
- Plenge P, Wiborg O. High-and low-affinity binding of S-citalopram to the human serotonin transporter mutated at 20 putatively important amino acid positions. Neurosci Lett 2005;383:203-8.
- Hoffman BJ, Mezey E, Brownstein MJ. Cloning of a serotonin transporter affected by antidepressants. Science 1991;254:579-80.
- Plenge P, Mellerup ET. An affinity-modulating site on neuronal monoamine transport proteins. Pharmacol Toxicol 1997;80:197-201.