High density lipoprotein 3 inhibits oxidized low density lipoprotein-induced apoptosis via promoting cholesterol efflux in RAW264.7 cells1
Introduction
Apoptosis is a key event in the progression of advanced atherosclerosis. Apoptosis of foam cells leads to the development of the necrotic core of an atherosclerotic plaque, and reduction of the excretion of cell matrix in the plaque region, which may contribute to plaque instability and the majority of cardiovascular complications[1–4]. Several lines of evidence strongly suggest that oxidized low density lipoproteins (ox-LDL) cause apoptosis and necrosis of macrophage cells[5,6]. After they are taken up by scavenger receptor A, ox-LDL inhibit the efflux of cholesterol from cells, resulting in the accumulation of a large amount of cholesterol within them[7–10]. Accumulating evidence suggests that elevation of cellular cholesterol plays a critical role in the regulation of ox-LDL-mediated cell apoptosis[11–14]. Thus, accelerating cholesterol efflux or preventing the accumulation of excess cholesterol in cells may inhibit ox-LDL-induced apoptosis, which could provide new perspectives for the prevention and reversal of atherosclerosis.
Having low levels of high density lipoprotein (HDL) cholesterol is an important cardiovascular risk factor. The atheroprotective activity of HDL is often attributed to its unique ability to facilitate the efflux of cholesterol from non-hepatic cells and subsequently deliver to the liver and steroidogenic organs, a process known as reverse cholesterol transport (RCT)[15,16]. Previous studies have revealed that HDL suppresses tumor necrosis factor α (TNF-α)-mediated apoptosis in cultured human endothelial cells[17]. However, whether HDL has a protective effect against ox-LDL-induced apoptosis of macrophages and whether this effect correlates with RCT has not yet been investigated. β-Cyclodextrins (β-CD) are cyclic oligomers of glucose that have the capacity to sequester cholesterol in their hydrophobic core. Recent studies have shown that β-CD is capable of stimulating efficient cholesterol efflux from cultured human fibroblasts[18]. In addition, brefeldin A (BFA), an antibiotic, has been shown to inhibit HDL-mediated cholesterol efflux from cholesterol-enriched cells[19]. The main aim of the present study was to observe whether HDL3 antagonized ox-LDL-induced apoptosis by promoting cholesterol efflux in RAW264.7 cells.
Materials and methods
Reagents Dulbecco’s modified Eagle’s medium (DMEM) and fetal bovine serum were purchased from Gibco/BRL (Grand Island, USA). A Hoechst 33258 staining kit was purchased from the Beyotime Institute of Biotechnology (Jiangsu, China). HDL3 was purchased from Huanan Company (Guangzhou, China). RAW264.7 cells (a murine macrophage cell line) were purchased from the Institute of Biochemistry and Cell Biology (Shanghai Institute for Biological Science, the Chinese Academy of Sciences, Shanghai, China). All reagents were of analytical grade.
Cell culture RAW264.7 cells were maintained in DMEM containing 10% fetal bovine serum in a humidified atmosphere of 5% CO2 and 95% O2. Cells were incubated with 50 mg/L ox-LDL for 48 h and then treated with various concentrations of HDL3 (50, 100, or 200 mg/L) or BFA (4 µmol/L) or β-CD (10 mmol/L) for 24 h before analysis. Untreated cells were used as controls.
LDL isolation and oxidization LDL was isolated from nonfrozen human plasma according to the method described in our previous report[20]. The remaining sample in the low-density fraction after isolation of very low-density lipoproteins was aliquoted into Quickseal tubes (Brown Caskets, Inc Miami, USA). The volume and the density of solution in each tube were adjusted to 35 mL and 1.063 kg/L with 38% NaBr and 0.15 mol/L NaCl, respectively. Tubes were sealed and centrifuged at 129 400×g at a temperature of 8 °C for 20 h. A sample of approximately 5 µL was recovered from superna-tant. The isolated LDL was oxidized with CuSO4 (10 µmol/L) for 18 h at 37 °C. The degree of oxidation of ox-LDL was assessed on the basis of increased mobility in agarose gel (compared with native LDL) and an increased level of thio-barbituric acid-reactive substances.
Flow cytometry Cultured RAW264.7 cells were collected and washed twice with phosphate-buffered saline (PBS). This was followed by fixing with cold 70% ethanol at 4 °C overnight and resuspending the cells in PBS. Cells were incubated with RNase A for 45 min and then stained with 50 mg/mL propidium iodide in the dark at 4 °C for 60 min. The suspension was analyzed with a FACScan flow cytometer (EPICS-XL, Beckman Coulter, Fullerton, USA). The apoptotic rate was determined on the basis of the “sub-G1” peak.
Hoechst 33258 staining Cells were incubated with or without ox-LDL and then harvested. After being fixed with 4% paraformaldehyde for 30 min at 25 °C, the preparations were washed with cold PBS 3 times and exposed to 10 mg/L Hoechst 33258 in the dark at room temperature for 10 min. Stained cells were observed under a fluorescence microscope. The cells with condensed chromatin and shrunken nuclei were counted as apoptotic cells.
High performance liquid chromatography analysis Cells were scraped from the culture flasks into 0.9% NaCl (1 mL per 50 cm2 flask) and homogenized on ice by sonication for 10 s. After protein concentration was determined by using a BCA kit (Pierce Biotechnology, Inc Rockford, USA), an equal volume of freshly prepared cold (-20 °C) KOH in ethanol (150 g/L) was added to cell lysates, and the mixture was repeatedly vortexed until clear. An equal volume of 3:2 hexane-isopropanol (v/v) was then added. The mixture was vortexed for 5 min, followed by centrifugation at 800×g (15 °C) for 5 min. The extraction procedure was repeated twice. The combined organic phase was transferred to clean tapered glass tubes and thoroughly dried under nitrogen at 40 °C. The tubes were allowed to cool to room temperature. One hundred microliters of isopropanol-acetonitrile 20:80 (v/v) was added. The sample was solubilized in an ultrasound water bath at room temperature for 5 min. After centrifugation at 800×g for 5 min, the samples were introduced into the high performance liquid chromatography (HPLC) device(Agilent 1100, Agilent Technologies, Inc., Palo Alto, USA). Cholesterol was eluted with 1 mL/min of eluent consisting of 20:80 isopropanol-acetonitrile (v:v) and detected by ultraviolet absorption at 206 nm[21]
Assessment of cholesterol efflux Cells were treated with [3H]cholesterol and cholesterol-loaded ox-LDL (50 mg/L) for 48 h. After being labeled with [3H]cholesterol, cells were washed and incubated for an additional 24 h in media containing bovine serum albumin (2 g/L) to allow for equilibration of [3H]cholesterol with intracellular cholesterol. Cells were then incubated with various concentrations of HDL3 (50, 100, and 200 mg/L), or BFA (4 µmol/L) or β-CD (10 mmol/L) in serum-free media. Media were recovered 24 h later. Cells were dissolved in N-2-hydroxyethylpiperazine-N'-2-ethane-sulfonic acid (HEPES; 1 mmol/L, pH 7.5) containing 0.5% Triton X-100. Media were briefly centrifuged to remove nonadherent cells. Aliquots of both supernatants and cells were then subjected to scintillation (FJ-2107P type liquid scintillator, Xi’an Nuclear Instrument Factory, Xi’an, China) for the determination of radioactivity. Cholesterol efflux was calculated by using the following formula: [3H]cholesterol in medium/([3H] cholesterol in medium+[3H]cholesterol in cells)×100%.
Statistical analysis Results are expressed as mean±SD. The two-tailed Student’s t-test was used for statistical comparisons. P<0.05 was considered to be statistically significant.
Results
ox-LDL induced apoptosis of RAW264.7 cells Exposure of RAW264.7 cells to 50 mg/L ox-LDL increased the apoptosis rate of cells in a time-dependent manner. The apoptotic rates after 24 h (23.1%) and 48 h (47.7%) treatment were both significantly higher than that in the control (5.14%; Figure 1).
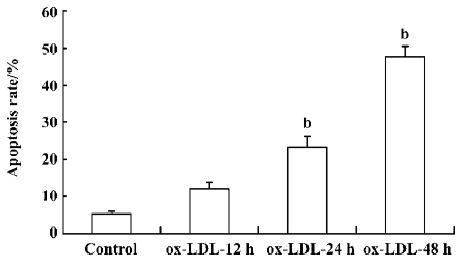
Effect of HDL3 on ox-LDL-induced apoptosis in RAW264.7 cells Typical apoptotic morphological changes, such as condensed chromatin and shrunken nuclei, were seen in RAW264.7 cells after the cells were incubated with ox-LDL for 48 h (Figure 2B). Co-administration of HDL3 inhibited these morphological changes (Figure 2C–2E) and decreased the apoptotic rate in a concentration-dependent manner (Figure 3).
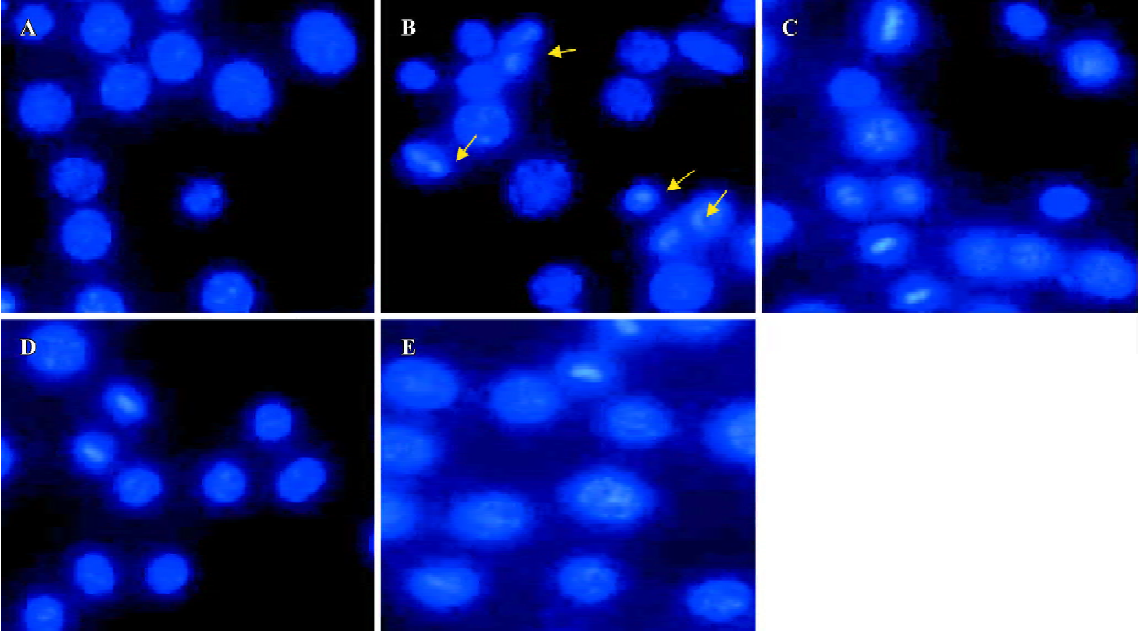
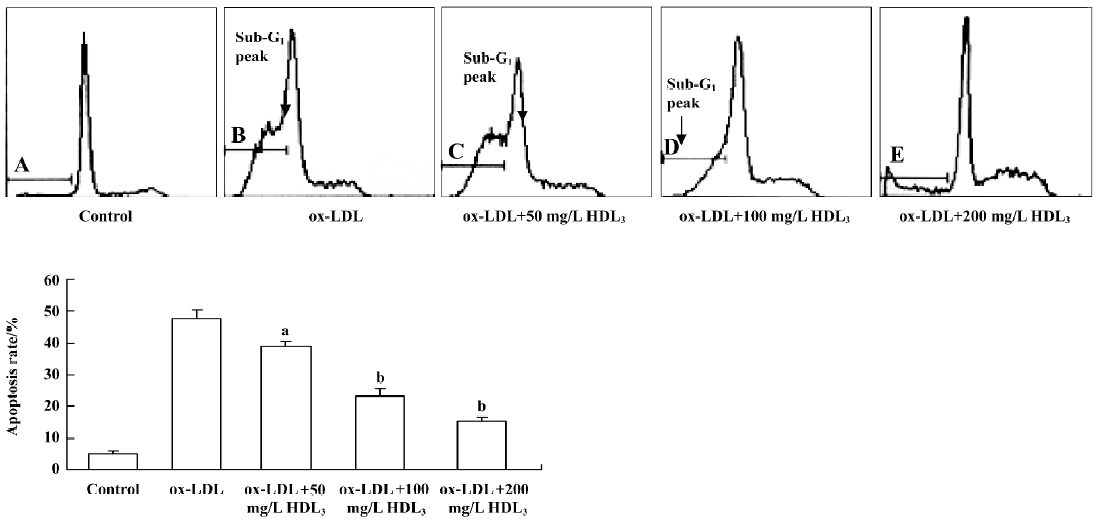
Effect of HDL3 on cholesterol efflux and cholesterol level in RAW264.7 cells Exposure of RAW264.7 cells to ox-LDL for 48 h increased total cholesterol from 153.4 mg/g protein to 505.1 mg/g protein without significantly affecting the rate of cholesterol efflux (Table 1). Many lipid droplets were seen after ox-LDL treatment (Figure 4B). Co-treatment with HDL3 and ox-LDL increased the rate of cholesterol efflux (Table 1), and decreased the total cholesterol (Table 1) and lipid droplets in cells (Figure 4C–4E). Both alterations were concentration-dependent (Table 1, Figure 4).
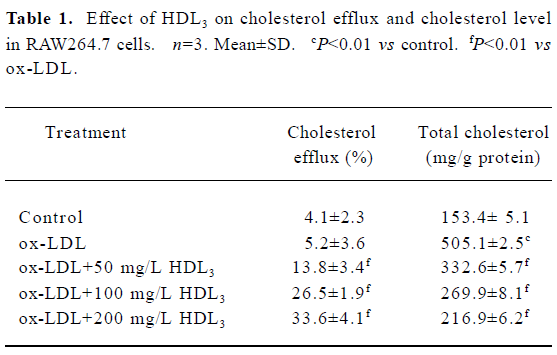
Full table
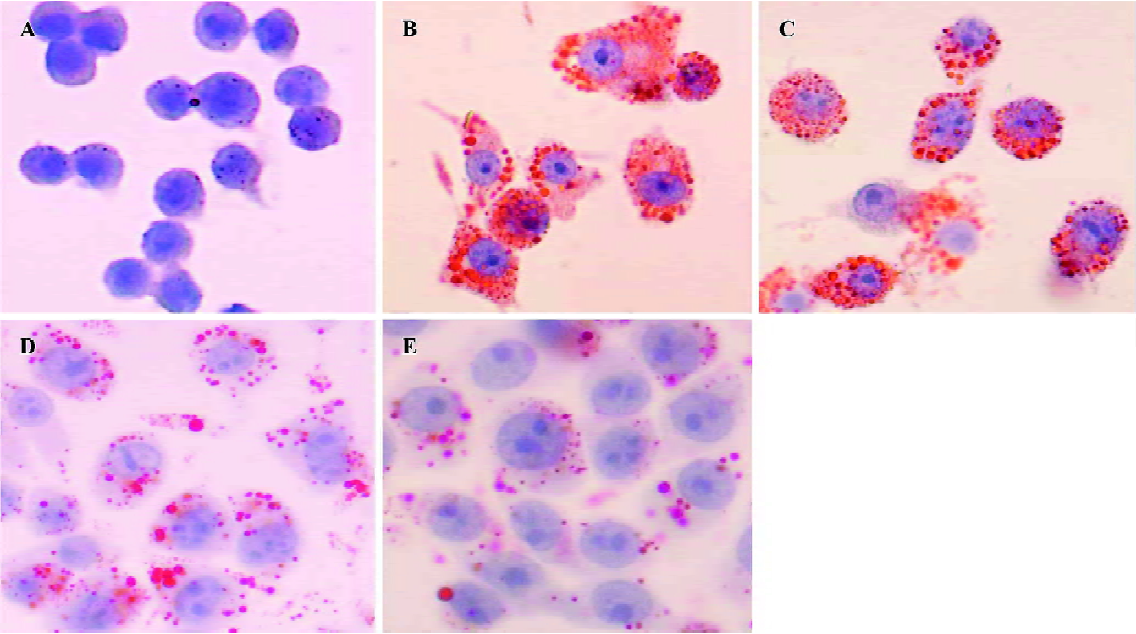
Effect of BFA and β-CD on apoptosis and cholesterol efflux in RAW264.7 cells Further observations with a blocker (BFA) and an agonist (β-CD) of cholesterol efflux were performed to investigate the relationship between HDL3 and reverse cholesterol transport. Flow cytometry analysis showed that BFA alone had no effect on cell apoptosis (Table 2). However, both the inhibition of ox-LDL-induced apoptosis (Table 2) and the facilitation of cholesterol efflux (Figure 5) by 100 mg/L HDL3 were significantly attenuated by BFA. Cholesterol acceptor β-CD decreased the ox-LDL-induced apoptotic rate from 47.7% to 14.2% (Table 2) and increased the rate of cholesterol efflux from 5.2% to 36.5% (Figure 5).
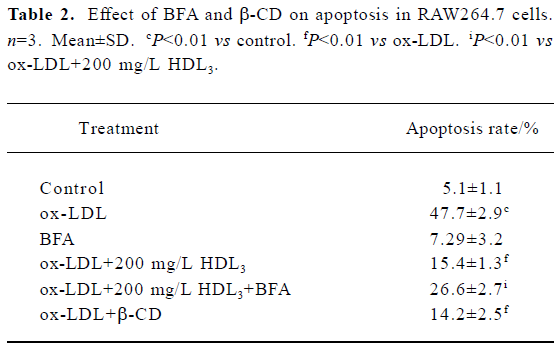
Full table
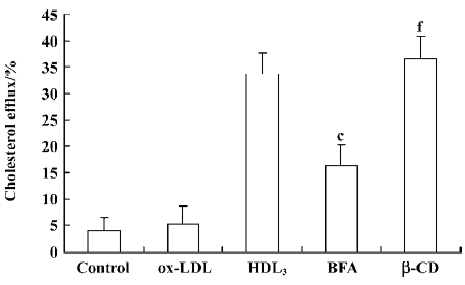
Discussion
Apoptosis is a prominent feature of atherosclerotic lesions in humans and experimental animals. It occurs in macrophages, T lymphocytes, and smooth muscle cells of the lesions, and is implicated in the development and progression of the disease. Increasing evidence suggests that apoptosis of foam cells, mostly macrophages that accumulate large quantities of free cholesterol, contribute to the expansion of a lipid core, and the large eccentric lipid core could lead to plaque instability by conferring a mechanical disadvantage on the plaque through redistribution of circumferential stress to the shoulder regions of the plaque, where nearly 60% of plaque ruptures tend to occur[1–4]. Therefore, inhibition of macrophage apoptosis may prevent the development of atherosclerosis.
Previous studies have shown that large amounts of cholesterol accumulate in macrophages treated with ox-LDL. Such a change in cholesterol leads to the failure of lipid homeostasis[10]. Furthermore, cellular accumulation of excess cholesterol may serve as a trigger for cell death, and promote ongoing inflammation, calcification, thrombosis and plaque rupture, which are the major sequelae of advanced atherosclerosis. HDL has been shown to protect cultured human endothelial cells induced by TNF-α through inhibition of caspase 3 and caspase 9 activity, and cytochrome c release[17,22]. However, whether HDL could protect monocyte-derived macrophage cells against ox-LDL-induced apoptosis has not yet been studied. Our data demonstrate that after incubation with 50 mg/L ox-LDL for 48 h, RAW264.7 cells display the morphological changes that are typically associated with apoptosis, such as condensed chromatin and shrunken nuclei, and up to 47.7% of cells are apoptotic. Treatment with HDL3 after 48 h pre-incubation with ox-LDL, decreased the apoptotic rate in a dose-dependent manner, and the apoptotic morphological changes were not present.
Cholesterol accumulation plays a critical role in the occurrence of ox-LDL-mediated cell apoptosis. Evidence suggests that accumulation of intracellular free cholesterol can increase Bax expression, increase cholesterol overloading of mitochondria, increase cytochrome c release, activate caspase-9, and lead to macrophage apoptosis[11–14]. Studies using experimental models of atherosclerosis indicate that the pro-apoptotic effect of ox-LDL can be reversed by lipid lowering interventions[23]. It is thus possible to protect cells from apoptosis by promoting intracellular cholesterol efflux. Efflux of cholesterol from extrahepatic tissue facilitated by HDL is considered to be an important mechanism by which HDL exerts its protective effects in atherosclerosis progres-sion. HDL enhances not only the translocation of cholesterol from intracellular compartments to the plasma membrane, but also cholesterol efflux from the plasma membrane to the extracellular space[24].
Various HDL subclasses are characterized by difference in shape, density, size, charge, and antigenicity. Mature HDL3 and HDL2 are generated from their precursors, lipid-free apoA-1 and lipid-poor pre-β1-HDL. Small HDL3 particles have a greater capacity to facilitate the efflux of cholesterol than large HDL2 because their small size makes it easier to move to the plasma membrane and bind to receptors (SR-B1) and cholesterol transporters (ABCA1). Furthermore, HDL3 exerts more powerful anti-oxidative effects than HDL2[25–29]. However, whether the protective effect of HDL3 on ox-LDL-induced apoptosis is mediated via the promotion of cholesterol efflux remains unclear. In line with this notion, we found that HDL3 dose-dependently increased the rate of cholesterol efflux, and significantly decreased the apoptotic rate of ox-LDL-treated RAW264.7 cells. Interestingly, when BFA, an antibiotic drug, was present at a concentration of 4 µmol/L during incubation of the cells with HDL3, the protective effect of HDL3 on ox-LDL-induced apoptosis was significantly attenuated. It has previously been shown that BFA inhibits HDL-mediated cholesterol efflux from cholesterol-enriched cells by disturbing the translocation of cholesterol from intracellular compartments to the plasma membrane[19]. Consistent with this finding, our data also revealed that BFA treatment decreased the rate of cholesterol efflux, and increased the level of total cholesterol, accompanied by an increase in the apoptotic rate of cells. In contrast, treatment with cholesterol acceptor β-CD, a cholesterol efflux stimulator, suppressed ox-LDL-induced apoptosis. Overall, our results suggest that promoting cholesterol efflux has great potential in the inhibition of ox-LDL-induced apoptosis in RAW264.7 cells.
In conclusion, cholesterol efflux mediates the protective effects of HDL3 on ox-LDL-induced apoptosis in RAW264.7 cells. This finding provides new evidence that HDL has a functionally protective effect on atherosclerosis and related complications.
Acknowledgements
The authors are indebted to Dr De-liang CAO (South Illinois University, USA) and Dr Ying-jun CAO (Johns Hopkins University, USA) for their critical discussion, suggestions, and valuable help in the preparation of the manuscript.
References
- Littlewood TD, Bennet MR. Apoptotic cell death in athero-sclerosis. Current Opin Lipidol 2003;14:469-75.
- Stoneman VE, Bennett MR. Role of apoptosis in atherosclerosis and its therapeutic implications. Clin Sci 2004;107:343-54.
- Kolodgie FD, Narula J, Haider N. Apoptosis in atherosclerosis. Does it contribute to plaque instability? Cardiol Clin 2001;19:127-39.
- Best PJM, Hasdai D, Sangiorgi G, Schwartz RS, Holmes DR, Simari RD, et al. Apoptosis. Basic concepts and implication in coronary artery disease. Arterioscler Thromb Vasc Biol 1999;19:14-22.
- Kinscherf R, Claus R, Wagner M, Gehrke C, Kamencic H, Hou D, et al. Apoptosis caused by oxidized LDL is manganese superoxide dismutase and p53 dependent. FASEB J 1998;12:461-7.
- Wintergerst ES, Jelk J, Rahner C, Asmis R. Apoptosis induced by oxidized low density lipoprotein in human monocyte-derived macrophages involves CD36 and activation of caspase-3. Eur J Biochem 2000;267:6050-9.
- Toru K, Kume N, Minami M, Hayashida K, Murayama T, Sano H, et al. Role of oxidized LDL in atherosclerosis. Ann NY Acad Sci 2001;947:199-206.
- Winther MP, Dijk KW, Havekes LM, Hofker MH. Macrophage scavenger receptor class a: a multifunctional receptor in athero-sclerosis. Arterioscler Thromb Vasc Biol 2000;20:290-7.
- Dhaliwal BS, Steinbrecher UP. Cholesterol delivered to macrophages by oxidized low density lipoprotein is sequestered in lysosomes and fails to efflux normally. J Lipid Res 2000;41:1658-65.
- Yancey PG, Jerome WG. Lysosomal sequestration of free and esterified cholesterol from oxidized low density lipoprotein in macrophages of different species. J Lipid Res 1998;39:1349-61.
- Tabas I. Consequences of cellular cholesterol accumulation: basic concepts and physiological implications. J Clin Invest 2002;110:905-11.
- Weibel GK, Jerome WG, Small DM, Warner GJ, Stoltenborg JK, Kearney MA, et al. Effects of intracellular free cholesterol accumulation on macrophage viability: a model for foam cell death. Arterioscler Thromb Vasc Biol 1998;18:423-31.
- Yao PM, Tabas I. Free cholesterol loading of macrophages is associated with widespread mitochondrial dysfunction and activation of the mitochondrial apoptosis pathway. J Biol Chem 2001;276:42468-76.
- Yao PM, Tabas I. Free cholesterol loading of macrophages induces apoptosis involving the Fas pathway. J Biol Chem 2000;275:23807-13.
- Rader DJ. High-density lipoproteins and atherosclerosis. Am J Cardiol 2002;90:62-70.
- Eckardstein AV, Nofer JR, Assmann G. High-density lipoproteins and arteriosclerosis: role of cholesterol efflux and reverse cholesterol transport. Arterioscler Thromb Vasc Biol 2001;21:13-27.
- Sugano M, Tsuchida K, Makino N. High-density lipoproteins protect endothelial cells from tumor necrosis factor-alpha-induced apoptosis. Biochem Biophys Res Commun 2000;272:872-6.
- Yancey PG, Rodrigueza WV, Stoudt GW, Johnson WJ, Philips MC, Rothblat GH. Cellular cholesterol efflux mediated by cyclodextrins. J Biol Chem 1996;271:16026-34.
- Mendez AJ. Monensin and Brefeldin A inhibit high density lipoprotein-mediated cholesterol efflux from cholesterol-enriched cells. J Biol Chem 1995;270:5891-900.
- Li LX, Chen JX, Liao DF, Yu L. Probucol inhibits oxidized-low density lipoprotein induced adhesion of monocytes to endothelial cells by reducing p-selectin synthesis in vitro. Endothelium 1998;6:1-8.
- Cullen P, Fobker M, Tegelkamp K, Meyer K, Kannenberg F, Cignarella A, et al. An improved method for quantification of cholesterol and cholesteryl esters in human monocyte-derived macrophages by high performance liquid chromatography with identification of unassigned cholesteryl ester species by means of secondary ion mass spectrometry. J Lipid Res 1997;38:401-9.
- Nofer JR, Levkau B, Wolinska I, Junker R, Fobker M, von Eckardstein A, et al. Suppression of endothelial cell apoptosis by high density lipoproteins (HDL) and HDL-associated lysosphingo-lipids. J Biol Chem 2001;276:34480-5.
- Martinet W, Knaapen MW, De Meyer GR, Herman AG, Kockx MM. Oxidative DNA damage and repair in experimental atherosclerosis are reversed by dietary lipid lowering. Circ Res 2001;88:733-9.
- Rothblat GH, Atger V, Kellner-Weibel G, Williams DL, Phillips MC. Cell cholesterol efflux: integration of old and new observations provides new insights. J Lipid Res 1999;40:781-96.
- Rye KA, Clay MA, Barter PJ. Remodeling of high density lipoproteins by plasma factors. Atherosclerosis 1999;145:227-38.
- Attie A, Kastelein JP, Hayden MR. Pivotal role of ABCA1 in reverse cholesterol transport influencing HDL levels and susceptibility to atherosclerosis. J Lipid Res 2001;42:1717-26.
- Tall AR, Costet P, Wang N. Regulation and mechanisms of macrophage cholesterol efflux. J Clin Invest 2002;110:899-904.
- Oram JF, Albers JJ, Cheung MC, Bierman EL. The effects of subfractions of high density lipoprotein on cholesterol efflux from cultured fibroblasts. Regulation of low density lipoprotein receptor activity. J Biol Chem 1981;256:8348-56.
- Anatol K, Sandrine C, Chapman MJ. Small, dense HDL particles exert potent protection of atherogenic LDL against oxidative stress. Arterioscler Thromb Vasc Biol 2003;23:1881-8.