Intramuscular delivery of rAAV-mediated kallikrein gene reduces hypertension and prevents cardiovascular injuries in model rats1
Introduction
Tissue kallikrein belongs to the serine proteinase family that cleaves a low molecular weight kininogen substrate to produce a vasodilator kinin peptide[1]. The binding of kinin to the bradykinin (BK) B2 receptor activates secondary messengers in target tissues and triggers a wide spectrum of biological effects, such as vasodilation, vasoconstriction, increase in vascular permeability, and the inhibition or stimulation of cell growth[2–5]. The vasodilatory action of the kallikrein-kinin system counterbalances the vasoconstrictive action of the renin-angiotensin system (RAS). Because of these functions, human tissue kallikrein (HK) has been explored for hypertensive gene therapy[5–7]. Several studies have demonstrated that the muscle delivery of HK gene can modulate blood pressure and ameliorate the secondary syndromes associated with hypertension in rats[8–12].
The recombinant adeno-associated virus (rAAV) vector is a promising for gene therapy. It can direct long-term transgene expression in many tissues, including the liver and muscles, in many animal studies. Since the adeno-associated virus (AAV) itself has not yet been found to be associated with any known human diseases, it is presumably safe for clinical use. In both animal experiments and clinical trials, rAAV vectors exhibited low vector toxicity. The prolonged transgene expression via persistent episomal concatamers also alleviates the fear of random insertion mutagenesis in the target cells.
Our previous study demonstrated that the systemic delivery of the rAAV vector-mediated HK gene resulted in stable, long-term expression and a sequential marked decrease in systolic blood pressure. It also attenuated organ damage due to hypertension in spontaneously hypertensive rats (SHR)[13]. Although intravenous rAAV delivery allowed for the efficient transduction and secretion of the target gene product, the systemic delivery of the AAV vector into the liver may not be an ideal method due to inherited risks. In a recent clinical trial with hemophilia B, it was found that liver complications could lead to a disappearance of the transgene expression. Because HK is a secretary enzyme protein that is secreted by cells into plasma or tissue fluid and can function systemically, we explored the possibility of delivering AAV–HK via intramuscular administration. In this study, we demonstrated that local rAAV-HK administration can lead to a long-term, stable expression of the HK protein and a stable reduction in the blood pressure of SHR, as reported in our previous study via intravenous delivery[13]. The results of the present study suggest that skeletal muscles may be a promising HK-producing organ for gene therapy.
Materials and methods
Plasmid construction The rAAV vector plasmid pXXUF1, the rAAV plasmid carrying LacZ as a report gene pdxII-LacZ, adenovirus helper plasmid pXX6, and packaging plasmid pXX2, were described previously[14]. The pXXUF1 contains 2 AAV inverse terminal repeats, a cytomegalovirus (CMV) promoter, and a poly A sequence. The plasmid pXX6 was constructed by inserting the large ClaI/SalI fragment of pXX5 into plasmid pBluescript KS (+). An 860 base pair NotI fragment containing the HK gene open reading frame was subcloned into pXXUF1 in the downstream of the CMV promoter to obtain plasmid pUF1-HK, which is used for the production of the rAAV vector expressing the HK gene.
rAAV vector production, purification, and titer determination For the rAAV production, human 293 cells were grown in Dulbecco’s modified Eagle’s medium (DMEM, Gibco, Grand Island, NY) supplemented with 10% fetal bovine serum (FBS, Gibco, USA) and antibiotics in a humidified environment with 5% CO2 at 37 °C. At 1–2 h prior to transfection, the cells (70%–80% confluent) were transfected with fresh DMEM containing 10% FBS. A total of 85 µg plasmid DNA/15 cm dish (molar ratio of pUF1-HK or pdxII-LacZ:pXX2:pXX6 was 1:1:1) was added to CaCl2 solution (0.25 mol/L) and then gently mixed with 2×BES-buffered saline (pH 7.0). The resulting DNA/calcium/phosphate complex was incubated at room temperature for 30 min before being applied to the 293 cells. At 8–12 h after transfection, the transfection mixture was replaced with fresh DMEM containing 10% FBS. The cells were harvested at 48–72 h postinfection. After low-speed centrifugation on a tabletop centrifuge, the cell pellets were resuspended in 100 mmol/L NaCl–10 mmol/L Tris-HCl (pH 8.5) and subjected to freeze-thaw for 4 cycles; the cell debris was removed by centrifugation. For a typical large-scale rAAV preparation, approximately 2×108 293 cells (40 15 cm dishes) were used for the transfection. For the purification, we adopted the single-step gravity-flow column purification procedure, which was described by Auricchio et al[15]. The eluted rAAV was aliquoted and stored at -80 ºC for vector administration.
To determine the titer for the rAAV-HK and rAAV-LacZ vectors, 5 µL rAAV was digested with 5 U DNase I at 37 ºC for 1 h. After DNase inactivation, 2×proteinase K buffer and 100 µg proteinase K were added and incubated with rAAV solution for 1 h at 37 °C, followed by extraction with an equal volume of phenol/chloroform/isoamyl alcohol. The resulting solution, which contained vector DNA, was then used for dot blot hybridization to determine the titers of rAAV-LacZ and rAAV-HK[16].
Animal handling and vector administration The 18 SHR were housed at room temperature with a 12 h light/dark cycle and allowed normal rat chow and tap water. All of the animal experimental protocols were approved by the Institutional Animal Research Committee of Tongji Medical College (Wuhan, China) and were carried out according to the guidelines of the National Institutes of Health. Two-month-old male SHR weighing 180–220 g were used for the gene delivery experiment. rAAV-HK or rAAV-LacZ (about 1×1011 viron particles in 1 mL saline solution) was gently injected into both sides of the femoral area of the SHR at 3 sites, and the control animals received an injection of equal saline volume (n=6 for each group). All the animals were killed 28 weeks after rAAV delivery under pentobarbital anesthesia (50 mg/kg body weight) and the rats’ hearts, lungs, kidneys, brains, aorta, and livers were collected, frozen in liquid nitrogen, and then stored at -80 ºC until further analyses. A portion of the organs was fixed with neutralizing formalin for the histological analysis.
Systolic blood pressure measurement The systolic blood pressure of the rats was measured with a manometer-tachometer (Rat tail NIBP system, ADI Instruments, Bella Vista, NSW, Australia) by cuffing the tails of the animals. This device requires minimal warming of rats (usually 15 min) before blood pressure determination and a brief period of restraint in a plastic cage. For every measurement, the systolic blood pressure was represented as the mean of at least 5 recordings.
Serum and urine collection The 24-h urine samples were collected in metabolic cages with 500 µL toluene to prevent the decay of urine. Approximately 1 mL blood was drawn from the tail vein, and sera were collected after coagulation and centrifugation. All samples were stored at -80 ºC before being assayed for kallikrein levels and the urinary microalbumin concentration.
Echocardiography analysis of heart etiology and cardiac function of SHR To perform the echocardiography analysis on the SHR, the animals underwent pentobarbital anesthesia (50 mg/kg body weight). Echocardiography took place at the imaging division of Tongji Hospital. The echocardio-graphy analysis was carried out by 2 echocardiogram experts independently.
Whole heart and left ventricle weight determination At 28 weeks’ post-HK gene delivery, the animals were killed; the hearts were collected and weighted. The left ventricle was weighed after the right ventricular free wall and both atrias were carefully dissected from the left ventricle. The inter-ventricular septum was included in the left ventricle for the weight measurement. The ratios of both whole heart/body weight and left ventricle/whole heart were then calculated.
RT-PCR analysis of HK mRNA Total RNA was extracted from fresh rat tissues using TRIZol reagent (Gibco, Carlsbad, CA, USA). RT-PCR analysis specific for HK mRNA was carried out using HK primers (sense: 5'-CCACCATGGGGTTCC-TGGTT-3' and antisense: 5'-CGCGGATCCACATTTGATTT-3'); the β-actin control primers were 5'-GGAGAAGATGACCC-AGATC-3' (sense) and 5'-GATCTTCATGAGGTAGTCAG-3' (antisense). RT-PCR was carried out following the instructions of the RT-PCR kit supplied by TaKaRa Biotechnology (Dalian, Liaoning, China). After first-strand cDNA synthesis, the PCR reaction was performed in the mixture at a final volume of 100 µL with 2 µL of the reverse transcription reaction product in a thermal cycler with a denaturing phase of 1 min at 94 oC, annealing phase of 40 s at 65 oC, and extension phase of 1 min at 72 oC for 20 cycles.
ELISA analysis of HK concentration The ELISA kit for HK in animal urine samples was obtained from Dr Chao LEE’s laboratory (Medical University of South Carolina, Charleston, SC, USA), and ELISA was performed according to the method described previously[8,13]. To make the biotin-labeled anti-HK immunoglobulin (IgG), purified rabbit anti-HK IgG was dialyzed against 0.1 mol/L sodium bicarbonate buffer, pH 9.5, at 4 °C for 24 h and added to 10 mL freshly prepared 0.1 mol/L biotinyl-N-hydroxy-succinimide ester (dissolved in dimethyl formamide). The reaction was carried out at room temperature for 1 h and the mixture was dialyzed against phosphate-buffered saline (PBS). Microtiter plates (96-well) were coated with non-labeled anti-HK IgG (2 µg/mL, 100 µL/well) overnight at 4°C. The plates were then blocked with 200 µL PBS (10 mmol/L sodium phosphate, pH 7.4, 150 mmol/L NaCl) containing 1% bovine serum albumin at 37 °C for 1 h. The coated plates were washed 3 times with PBS containing 0.1% Tween-20 (washing solution). The purified HK standard (0.04–2.5 µg) and rat urine or serum samples were added to individual wells in a total volume of 100 µL PBS containing 0.05% Tween-20 and 0.5% gelatin (dilution buffer). After incubation at 37 °C for 90 min, the plates were washed 3 times and 100 µL of 1 µg/mL biotin-labeled anti-HK IgG was added to each well. After incubation at 37 °C for 1 h, the plates were washed 3 times and 100 µL of 1 µg/mL peroxidase-avidin was applied to each well. The plates were subsequently incubated at 37 °C for 1 h and washed 5 times with the washing solution and once with PBS before adding 100 µL freshly-prepared substrate solution (0.03% 2,2'-azino-bis[3-ethyl benzthiazoline-6-sulfonic acid]; 0.03% H2O2 in 0.1 mol/L citrate buffer, pH 4.3). The plates were read at 405 nm on an ELX 800 ELISA reader (Bio-Tek instruments, Winooski, Vermont, USA).
Analysis of morphology and collagen deposition A portion of the kidney and heart of each animal were preserved in 4% PBS-buffered formaldehyde solution and embedded in paraffin. Four micrometer-thick sections were cut and stained with hematoxylineosin and Sirius Red (collagen stains red with Sirius Red staining) using the method described by Dobrzynski et al[17]. To evaluate and quantify the collagen density in the tissues, sections of the heart, kidney, and aorta were analyzed by using HAIPS pathological imagic analysis system after Sirius Red staining, and the results were expressed as the ratio of collagen to non-collagen area (percentage of extracellular matrix [ECM]).
Statistical analysis The statistical significance of the difference in systolic blood pressure among SHR receiving rAAV-LacZ, rAAV-HK, and saline, respectively, was determined by ANOVA. In addition, we used unpaired Student’s t-test to assess the differences in the urine microalbumin levels, HK levels in the urine and serum, the ratios of both whole heart/body weight and left ventricle/total heart, and the results of the echocardiography analysis between the rAAV-LacZ and rAAV-HK groups after gene delivery. The results were expressed as mean±SEM.
Results
HK expression detection in the muscles post-rAAV-HK intramuscular vector delivery To assess the expression of HK post-intramuscular AAV gene delivery, total cellular RNA was isolated from the heart, renal, lung, liver, and skeletal muscles at the femoral area at 28 weeks using TRIZol reagents. RT-PCR was performed using 5 µg total RNA and oligonucleotide primers designed according to the sequence of the HK gene. As shown in Figure 1, human kallikrein mRNA was detected in skeletal muscle tissues at the injection sites in rAAV-HK-treated rats, but not in other tissues in the HK group and all the tissues of the rAAV-LacZ-treated rats. A similar level of β-actin mRNA was detected in the tissues of both the experimental and control groups. This result suggests that the muscle tissue is the primary site for HK gene transcription and protein expression post-intramuscular rAAV gene delivery.
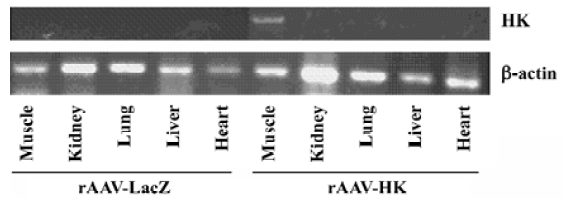
HK gene delivery leads to the secretion of immunoreactive HK We next examined the expression of HK in the urine and blood of the SHR by ELISA. As expected, no immunoreactive HK was detected in the urine of the control rats receiving rAAV-LacZ and saline. In contrast, immunoreactive kallikrein in the urine of rats receiving rAAV-HK vectors intramuscularly reached 4.86±0.347 ng/mL in 2 weeks and maintained at a similar level throughout the entire experimental period (Figure 2A). Correspondingly, HK in the serum of the testing group receiving rAAV-HK was maintained at a steady level of 1.12±0.068 ng/mL. It was parallel to the kallikrein levels in the urine in all the durations of the experiment although it was lower than that in the urine of the rats receiving rAAV-HK intravenously[13]. In the serum of the control animals receiving AAV-LacZ or PBS, only the basal level of kallikrein was detected, which was likely due to a cross-reaction of antihuman kallikrein antibodies (Figure 2B). The results demonstrate that rAAV-mediated intramuscular HK transfer can lead to the persistent and high level secretion of the HK protein in vivo.
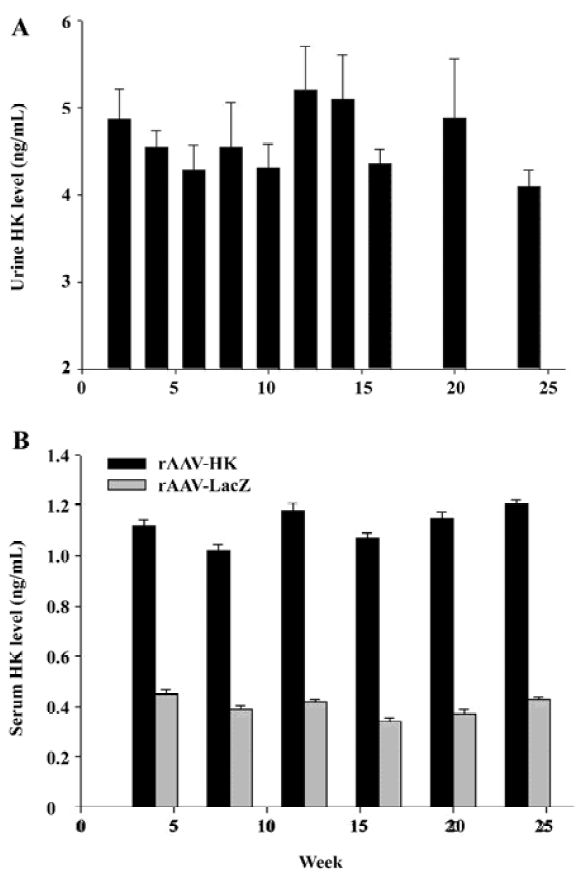
Antihypertensive effect of rAAV-HK in SHR To study the antihypertensive effect of rAAV-HK delivered intramuscularly, we monitored the systolic blood pressure of SHR receiving the control vector and rAAV-HK. In the control group receiving AAV-LacZ or saline, the systolic blood pressure of the rats kept rising and reached 180–185 mmHg at week 28 and then stabilized until the end of the experiments. In contrast, in the rAAV-HK-treated animals, blood pressure was lowered to 168–178 mmHg. The average difference between the rats receiving AAV-LacZ and AAV-HK was 12.6±1.93 mmHg (P<0.05, n=6, ANOVA). There was no significant difference between the LacZ- and saline-treated groups (Figure 3). These results indicate that the intramuscular delivery of rAAV-HK induced a stable and long-term hypotensive effect and prevented the development of hypertension in SHR.
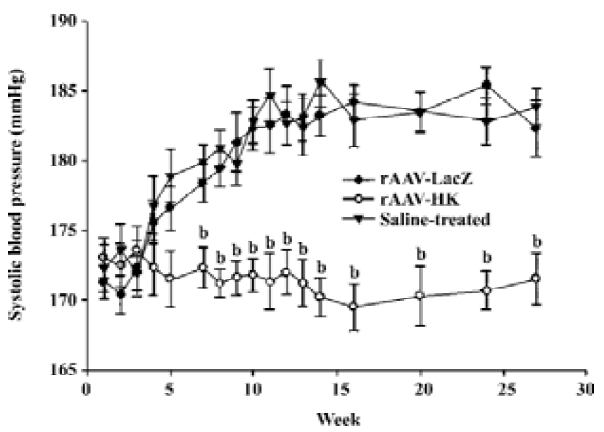
Protective effects of HK on kidney histology To analyze the secondary effects of the intramuscular delivery of rAAV–HK, we inspected the extent of kidney damage of the experimental groups by hematoxylin-eosin staining. As shown in Figure 4, hypertension induced severe damage in the kidneys of the control SHR, which included tubular dilatation, atrophy of partial renal tubules, loss of brush border in proximal tubules and the formation of protein casts, and glomerular sclerosis and atrophy as observed in other studies[18,19]. On the contrary, rAAV-HK delivery significantly attenuated this renal damage induced by hypertension. In the rAAV-HK-treated rats, the kidney structures, including the renal cortex and medulla, exhibited little pathological changes (Figure 4).
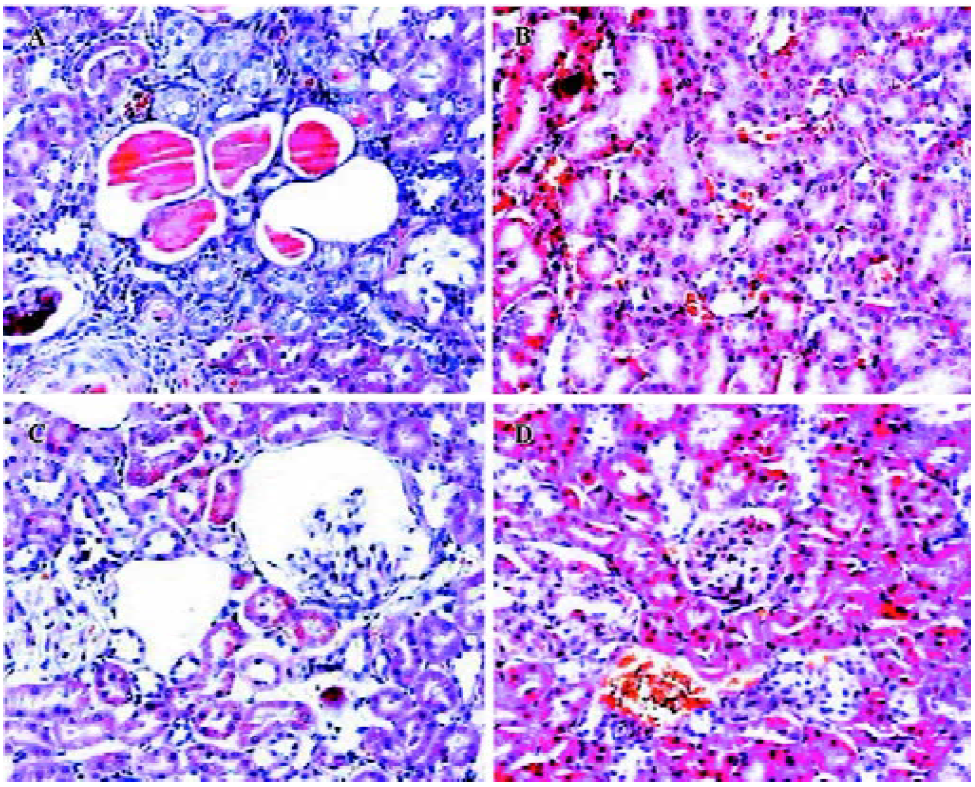
Fibrosis of the kidneys was then assessed using Sirius Red staining. To quantify ECM stained by Sirius Red, the red staining area was expressed as a percentage of the total tissue area. This percentage was used as an index of fibrosis. The results showed that many intense red staining in the glomerulus and medulla vascular areas in the control SHR, which represented the collagen deposition in the kidney. In contrast, the SHR treated with the HK gene showed much less collagen deposition or ECM accumulation than the LacZ-treated animals (10.06%±2.94% vs 24.77%±2.08%, P<0.05, n=6; Figure 5). This analysis suggests that HK attenuated the collagen deposition or fibrosis in the SHR kidney, which is the primary mechanism in which rAAV-HK treatment is able to protect the kidney from hypertensive injury.
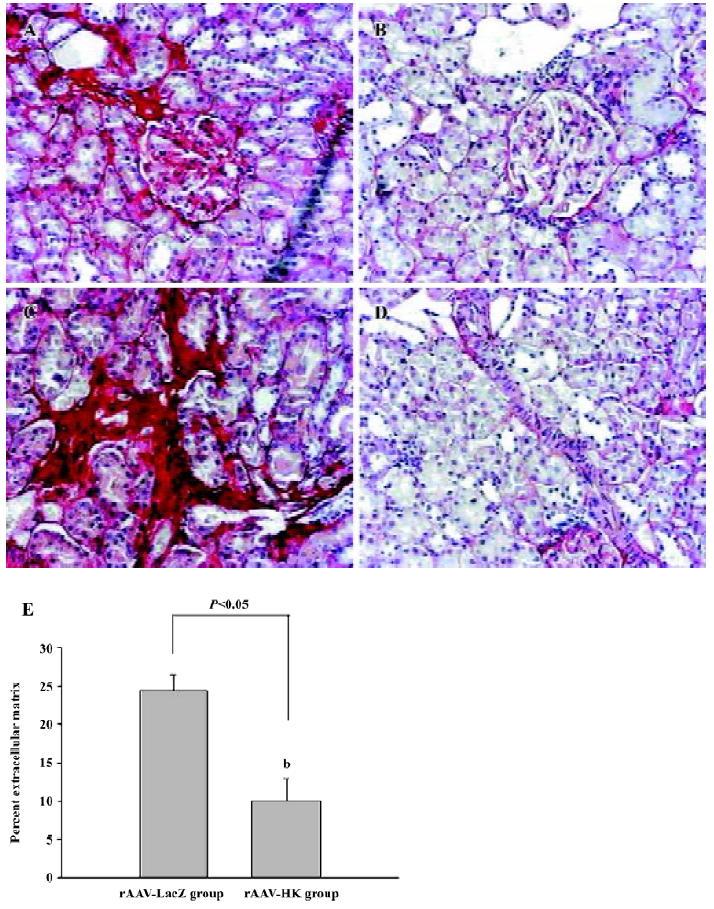
Attenuation of cardiovascular remodeling and cardiac hypertrophy Myocardial hypertrophy is an important part of heart injury resulting from hypertension as well as an independent predictor of cardiovascular events in clinical practice. It is also an important consideration in the therapy of the hypertension to reverse cardiac hypertrophy[20]. In this study, we analyzed the effects of rAAV-HK on hypertensive cardiac hypertrophy. The results are shown in Figures 6 and 7. First, the ratio of total heart weight to body weight in the rAAV-HK-treated SHR was significantly lower than the control SHR (4.378±0.0530 mg/g vs 5.100±0.0489 mg/g, P<0.05, n=6). The rAAV-HK delivery also significantly attenuated the ratio of the left ventricle weight to whole heart weight compared with the control SHR (0.782±0.0163 g/g vs 0.834±0.0116 g/g, P<0.05, n=6; Figure 6). Second, the result of the echocardiography analysis for the rAAV-HK-treated rats showed the value of the interventricular septum thickness (IVS; 2.90±0.041 mm in HK rats vs 3.45±0.050 mm in control rats, P<0.05, n=6), and the percentage values of endocardial fractional shortening (FS%; 69.5%±0.646% vs 62.5%±1.50%, P<0.05, n=6) were obviously ameliorated compared with those in the control animals (Figure 7). These results indicated that the heart structure and cardiac function in the experimental group were significantly more attenuated than the control groups. Furthermore, a microscopy observation after Sirius Red staining showed that the sizes of the cardiac myocytes were not uniform. The structures were deranged and there was a red-stained area in the left ventricle section of the heart in the control rats. This indicated significant fibrosis in the myocardium. rAAV-HK treatment significantly alleviated cardiac myocyte hyper-trophy, derangement of structures, matrix prolifera£tion, and cardiac fibrosis. ECM accumulation expressed as the percentage of collagen per total cardiac tissue section was less than that of the control animals (3.40%±2.03% vs 19.55%±5.20%, P<0.05, n=6; Figure 8). Finally, rAAV-HK treatment significantly prevented aorta fibrosis. The ECM accumulation percentage per total aorta tissue section in the HK group was 19.78%±1.34% compared to 26.26%±1.28% in the rAAV-LacZ group (P<0.05, n=6).
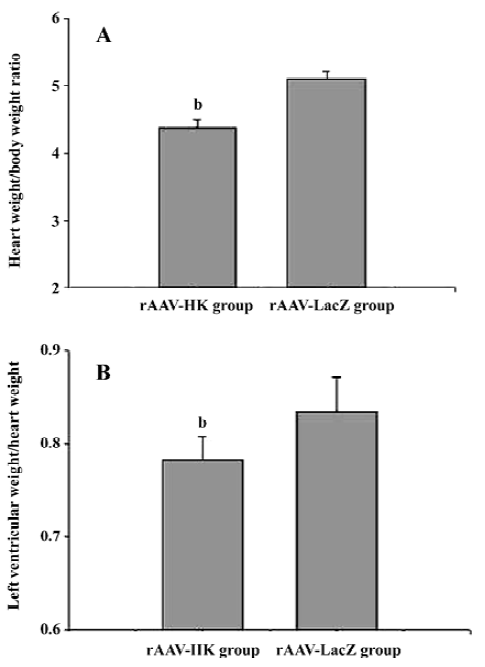
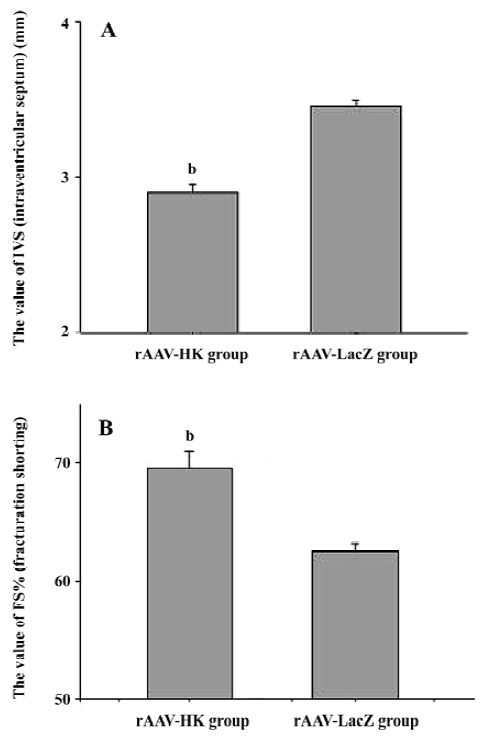
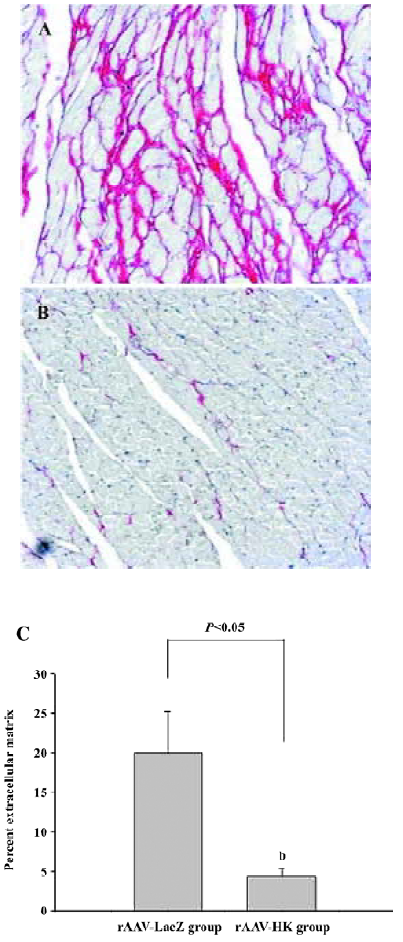
Discussion
In our previously published study, we documented that the intravenous administration of rAAV-HK into SHR resulted in the long-tern expression of the HK gene and a persistent reduction of blood pressure in rats; it also prevented organ damage secondary to hypertension, which suggests that rAAV-mediated HK gene delivery may act as a safe and effec-tive therapeutic approach in the treatment of hypertension or other cardiovascular and renal diseases. However, the concern is whether diverse infection of rAAV in various organs will induce any unexpected, adverse effects. Thus, local infection in skeletal muscles is a promising choice. In the present study, an equal dose of rAAV-HK was administered intramuscularly to SHR, resulting in a long-term and stable HK expression. Because tissue kallikrein is a secretary protein, a single intramuscular injection of AAV-HK, with same dose as the intravenous injection we previously administered[13], led to the persistent expression of HK, and comparable HK levels as shown by RT-PCR and the ELISA assay for HK. Similar to the results from the intravenous delivery study, intramuscularly-delivered HK attenuated the development of hypertension and prevented secondary damage in the target organs of young SHR. Cardiac hypertrophy, fibrosis, and dysfunction were reduced. Cardiac remodeling and dysfunction were confirmed by cardiac hemodynamic measurements and echocardiography. Moreover, the kidney was susceptible to hypertension. With rAAV-HK-mediated gene delivery, renal damage might be alleviated, which was confirmed by morphological observation and a quantity analysis of ECM or collagen deposition[13]. The results demonstrate that skeletal muscles may be a promising target organ for hypertension gene therapy.
The rAAV vector is one of the most attractive vectors for gene therapy because of its ability to direct a persistently high level of transgene expression in both dividing and non-dividing cells and low vector toxicity. To our knowledge, no report about the pathogenesis of the rAAV vector has been published[21]. One of the ideal targets for rAAV gene delivery is skeletal muscles, which is the largest organ in the human body, making up of about 40% body weight in adults. The metabolism of skeletal muscles is very active and its supplement of blood is abundant. All these features make this specific organ a promising gene transfection and transgenic protein-producing concourse[18,19,22,23]. Previous studies have documented that rAAV vectors allow efficient gene transfer into the muscles. Recombinant AAV encoding the dystrophy gene, human α1-antitrypsin, factor IX, and human erythropoietin gene, all successfully corrected their related phenotypes in the animal studies[24,25]. In the current studies, we demonstrated that the rAAV vector is a suitable vector for delivering another secretary protein HK to reduce hypertension and tissue organ pathogenesis associated with hypertension.
Tissue kallikrein is a serine proteinase which can produce vasodilative peptides, kinin, and BK[1]. The binding of kinins to the BK B2 receptor activates second messengers in target tissues and triggers a wide spectrum of biological effects, such as vasodilation and the inhibition or stimulation of cell growth[2–4]. The vasodilatory action of the kallikreinkinin system counterbalances the vasoconstrictive action of the RAS[26]. This is the primary reason why HK is effective in controlling hypertension. Since B2 receptors express abundantly in the kidney, aorta, and heart (unpublished data), it is likely that kinin and BK may directly activate the B2 receptor in these tissues and therefore attenuate cardiovascular and renal remodeling.
In conclusion, the intramuscular rAAV-HK gene delivery can result in therapeutic level of HK gene expression in vivo. The sustained alleviation of hypertension and target organ protection from hypertensive damage can also be achieved by a single dose of vector administration. Because the intramuscular administration of rAAV-HK has the same efficiency as intravenous rAAV-HK delivery, the skeletal muscles might be a promising organ for rAAV-HK gene delivery which should not be ignored. Thus, it warrants further studies to explore the full potential of rAAV-HK in treating hypertension.
References
- Kitamura NKH, Fukushima D, Takagaki Y, Miyata T, Nakaniski S. Structural organization of the human kininogen gene and a model for its evolution. J Biol Chem 1985;260:8610-7.
- Müller-Esterl W IS, Nakaniski S. Kininogens revisited. Trends Biochem Sci 1986;11:336-9.
- Nakaniski SSP. Precursor and kininogen: their structures, gene organizations, and regulation. Physiol Rev 1987;67:1117-42.
- Adam AAA, Caloy G, Closset J, Damas J, Franchiment P. Human kininogens of low and high molecular mass: quantification by radioimmunoassay and determination of reference values. Clin Chem 1985;31:423-6.
- harma JNUK, Noor AR, Rahman AR. Blood pressure regulation by the kallikrein-kinin system. Gen Pharmacol 1996;27:55-63.
- Katori MMM. Pivotal role of renal kallikrein-kininsystem in the development of hypertension and approaches to new drugs based on this relationship. Jpn Pharmacol 1996;70:95-128.
- Margolius HS. Kallikreins and kinins: some unanswered questions about system characteristics and roles in human disease. Hypertension 1995;26:221-9.
- Xiong WCJ, Chao L. Muscle delivery of human kallikrein gene reduces blood pressure in hypertensive rats. Hypertension 1995;25:715-9.
- Murakami HYK, Chao L, Chao J. Human kallikrein gene delivery protects against gentamycin-induced nephrotoxicity in rats. Kidney Int 1998;53:1305-13.
- Chao JZJ, Lin KF, Chao L. Human kallikrein gene delivery attenuates hypertension, cardiac hypertrophy and renal injury in Dahl salt-sensitive rats. Hum Gene Ther 1998;9:21-31.
- Chao JZJ, Lin KF, Chao L. Adenovirus-mediated kallikrein gene delivery reverses salt-induced renal injury in Dahl salt-sensitive rats. Kidney Int 1998;54:1250-60.
- Dobrzynski EYH, Chao J, Chao L. Adenovirus- mediated kallikrein gene delivery attenuates hypertension and protects against renal injury in deoxycorticosterone-salt rats. Immunopharma-cology 1999;44:57-65.
- Wang TL, Zhao C, Chen C, Li J, Chao J, Chao L, et al. Recombinant adeno-associated virus-mediated kallikrein gene therapy reduces hypertension and attenuates its cardiovascular injuries. Gene Therapy 2004;11:1342-50.
- Xiao X, Li J, Samulski RJ. Production of high-titer recombinant adeno-associated virus vectors in the absence of helper adenovirus. J Virol 1998;72:2224-32.
- Auricchio A, Hildinger M, O’Connor E, Gao G, Wilson JM. Isolation of highly infectious and pure adeno-associated virus type 2 vectors with a single-step gravity-flow column. Human Gene Therapy 2001;12:71-6.
- Gao GP, Qu G, Faust LZ, Engdahl RK, Xiao W, Hughes JV, et al. High-titer adeno-associated viral vectors from a Rep/Cap cell line and hybrid shuttle virus. Hum Gene Ther 1998;9:2353-62.
- Dobrzynski EMD, Agata J, Zhu J, Chao J, Chao L. Adrenomedullin improves cardiac function and prevents renal damage in streptozotocin-induced diabetic rats. Am J Physiol Endocrinol Metab 2002;283:E1291-E1298.
- MacColl GS, Goldspink G, Bouloux PM. Using skeletal muscle as an artificial endocrine tissue. J Endocrinol 1999;162:1-9.
- Danko I, Fritz JD, Jiao S, Hogan K, Latendresse JS, Wolff JA. Pharmacological enhancement of in vivo foreign gene expression in muscle. Gene Therapy 1994;1:114-21.
- Okin PM, Devereux RB, Jern S, Kjeldsen SE, Julius S, Nieminen MS, et al. Regression of electrocardiographic left ventricular hypertrophy during antihypertensive treatment and the prediction of major cardiovascular events. JAMA 2004;292:2343-9.
- Monahan PE SR. AAV vectors: is clinical success on the horizon? Gene Ther 2000;7:24-30.
- Liu F LK, Huang L. Systemic administration of naked DNA: gene transfer to skeletal muscle. Mol Interv 2001;1:168-72.
- Blau HM, Springer ML. Muscle-mediated gene therapy. N Engl J Med 1995;333:1554-6.
- Wang B, Li J, Xiao X. From the cover: adeno-associated virus vector carrying human minidystrophin genes effectively ameliorates muscular dystrophy in mdx mouse model. Proc Natl Acad Sci USA 2000;97:13714-9.
- Bohl D, Bosch A, Cardona A, Salvetti A, Heard JM. Improvement of erythropoiesis in beta-thalassemic mice by continuous erythropoietin delivery from muscle. Blood 2000;95:2793-8.
- Schmaier AH. The kallikrein-kinin and the renin-angiotensin systems have a multilayered interaction. Am J Physiol Regul Integr Comp Physiol 2003;285:R1-R13.