Ischemic preconditioning protects post-ischemic renal function in anesthetized dogs: role of adenosine and adenine nucleotides1
Introduction
Renal dysfunction is a serious and common complication of ischemia-reperfusion injury[1], and the onset of acute renal failure secondary to ischemia-reperfusion injury implies a poor prognosis and is frequently associated with a high mortality rate[2]. In animal and human models, it has been demonstrated that prolonged ischemia results in an immediate decrease in renal blood flow (RBF) and glomerular filtration rate (GRF)[3].
Recent developments in renal physiology have determined that rat kidneys can be ischemic preconditioned against ischemia-reperfusion injury[4]. A possible candidate mediator of ischemia-reperfusion injury is adenosine, which has been shown to be involved in ischemic-induced renal vasoconstriction via its effects on adenosine A1 receptors. Its antagonist has a partial protective effect against renal vasoconstriction during ischemia[5]. Extensive evidence exists demonstrating the beneficial effects of ischemic preconditioning (IPC) in cardiac muscle[6,7]. However, evidence supporting a role for IPC in protecting against ischemia-reperfusion injury in kidney is minimal and controversial[4,8,9]. Recently, Lee and Emala[4] demonstrated that IPC (4 cycles of 8-min renal ischemia followed by 5-min reperfusion) protected renal function after ischemia and reperfusion (45 min of renal ischemia followed by 24-h reperfusion). However, Islam et al[8] failed to demonstrate protective effects of renal IPC in rats (4 cycles of 4-min renal ischemia followed by 11-min reperfusion periods).
Lee and Emala[4] reported that in the rat kidney, adenosine or A1 receptor agonist infusions before ischemic insult protected renal function via A1 adenosine receptor activation. However, Sugino et al[10] reported that the adenosine A1 receptor antagonist failed to abolish the post-ischemic renal protective effects of IPC or exogenous adenosine in the rat kidney. The results of these experiments indicate that IPC appears to increase extracellular adenosine concentration, which in turn mimics post-ischemic renal function. Our previous study showed that renal interstitial adenosine[11] concentration significantly increased during ischemia. Adenosine has been demonstrated to elicit renal vasoconstric-tion[12–14]; it is suggested that extracellular adenosine could be involved in the mediation of post-occlusion renal ische-mia[15–17]. However, findings in the above literature are indirect and do not provide any data supporting the renal interstitial concentrations of adenosine and adenine nucleotides during IPC followed by ischemia and reperfusion. In addition, it is not clear if IPC protects against post-ischemic renal functions in the dog kidney. The beneficial effects of using multiple cycles over single cycle IPC, that is, cycles of multiple brief IPC, is correlative to its protective effect and is poorly understood. As well as this, the influence of IPC on the intrarenal hemodynamics is unclear.
The aim of the present study is to confirm the protective role of IPC on ischemia-reperfusion injury in dogs, and to determine that a correlation exists between the cycles of IPC and its protective effects. This study was also undertaken to determine the relationship between multiple brief ischemic preconditioning and the intrarenal hemodynamics of adenosine and adenine necleotide, including the role of the adenosine A1 receptor. In the present study, microdialysis sampling technology was introduced to measure the intrarenal hemodynamics as well as the dynamics of renal interstitial concentration of adenosine, adenine nucleotide during IPC and ischemia-reperfusion.
Materials and methods
General procedure All surgical and experimental procedures were performed according to the guidelines for the care and use of animals as established by the Kagawa Medical University. Adult mongrel dogs weighting 10–15 kg were used. They had been maintained on standard laboratory food for 1 week. The surgical preparation of the animals and basic experimental techniques are identical to those previously described[11,8]. The animals were anesthetized with sodium pentobarbital (0.03 g/kg, iv) and given additional doses as required. After tracheotomy, the animals were mechanically ventilated with Harvard apparatus ventilator(Harvard Apparatus, Millis, MA, USA). A polyethylene catheter was inserted into the right brachial vein for infusion of isotonic saline or drug solution. Isotonic saline or drug solution was infused at a rate of 0.2 mL⋅min-1⋅kg-1 throughout the experiment. Another catheter was placed in the abdominal aorta via the right femoral artery, and mean arterial pressure (MBP) was continuously measured and recorded with a pressure transducer (NEC-San-ei Model N
The left kidney was exposed through a retroperitoneal flank incision. The kidney was carefully denervated by dissecting all visible nerve fibers, as well as the tissue connecting the renal hilum cephalic, to the renal artery. An electromagnetic flowmeter (MFV-1200, Nihon Kohden, Tokyo, Japan) was positioned around the renal artery, and renal blood flow (RBF) was continuously monitored. A polyethylene catheter was inserted into the left ureter, and urine samples were continuously collected in tubes. The total of urine collected in every consecutive 10-min period was used to calculate urine flow (UF) rate (L/min). The renal capsule was carefully opened along the convexity, and the micro-dialysis probes were gently implanted into the renal cortex as shown by Nishiyama et al[11,19]. The probes were connected to a CMA/100 microinfusion pump (Carnergie Medicine, Stockholm, Sweden) and were perfused with isotonic saline solution with heparin (0.03 units/L) containing iodotubercidine (10 µmol), an inhibitor of adenosine kinase, and erythro-9-(2-hydroxy-3-nonyl)adenine (EHNA) (100 µmol), an inhibitor of adenosine deaminase, at a rate of 5 µL/min. The dialysate was collected in a chilled tube over a 5-min sample period and analyzed for adenosine concentration as well as inosine, hypoxanthine, xanthine, and uric acid concen-trations. Samples were stored at -70 °C prior to analysis. After surgery, the dogs were left alone for 90 min for the tissue to recover from the trauma produced by insertion of the probes and to allow for the stabilization of mean arterial pressure, renal blood flow, and renal interstitial adenosine concentration. At the end of each experiment, the exact position of the probe was verified by inspection. If the probe was situated in the outer medulla at least in part, we omitted the data of these dialysates.
Characteristics of microdialysis probe For the determination of renal interstitial concentrations of adenosine, inosine, hypoxanthine, xanthine, and uric acid, we used a microdialysis probe. The dialysis membrane is made from cuprophan fiber, measuring 15-mm in length with a 5500-Da transmembrane diffusion cut off (Toyobo, Otsu, Japan). Thin stainless steel tubes (outer diameter: 190 µm, inner diameter:100 µm) were inserted into both sides of the cuprophan fiber. The inflow and outflow ends were inserted into the polyethylene tubes (PE-10) and sealed in place with glue. Preliminary results from in vitro experiments demonstrated that a negligible amount of adenosine, inosine, hypoxanthine, xanthine, and uric acid would stick to the polyethylene tubes.
The efficiency of the microdialysis probe was determined as follows. Briefly, the probes were soaked in beakers containing saline solution with different concentrations (0.1–5 µmol/L) of adenosine, inosine, hypoxanthine, xanthine, and uric acid (n=26). The probes were perfused with saline solution with heparin (0.03 units/L) containing iodotubercidine (10 µmol) and EHNA (100 µmol) at a rate of 5 µL/min. The dialysates were collected, and the equilibrium rates for adeno-sine, inosine, hypoxanthine, xanthine, and uric acid were calculated by dividing the concentrations in the dialysates at the concentrations in the medium. At a perfusion rate of 10 µL/min, the relative equilibrium rates of adenosine, inosine, hypoxanthine, xanthine, and uric acid were 36.9%±1.9%, 39.7%±3.2%, 50.3%±4.1%, 46.9%±2.5%, and 34.7%±4.2%, respectively.
Experimental protocols
Effects of ischemic-preconditioning on renal hemodynamics and renal interstitial concentration of adenosine and adenine nucleotides Dogs were randomized to 60-min renal ischemia and 60-min reperfusion (No IPC) group, which were treated with the adenosine A1 receptor antagonist in advance, three ischemic preconditioning groups were treated with 1–3 cycles of 10-min renal ischemia and 10-min reperfusion, using an adjustable renal arterial clamp, respectively, followed by 60-min renal ischemia. During each ischemia-reperfusion interval, 5 min was allowed for stabilization before samples from all 5 probes used for measurements of adenosine, inosine, hypoxanthine, xanthine and uric acid were collected. After the initial stabilization period, the experimental protocols were started with renal interstitial adenosine and adenine nucleotides collections, and MBP, UF as well as RBF measurements for 2 consecutive 10-min periods. After collection or measurements of the second control sample, 60-min renal ischemia followed by 60-min reperfusion or IPC treatment were performed. At the same time, UF and RBF were also measured continuously.
Effects of ischemia-reperfusion on renal hemodynamics during treatment with KW3902 KW3902 (Kyowa Hakko Kogyo Co Ltd, Tokyo, Japan), a highly selective adenosine A1 receptor antagonist, was dissolved in the lactated Ringer’s solution containing 1% dimethylsulfoxide and 0.01 mol/L NaOH. After twice 10-min sampling periods, KW3902 (priming dose, 0.1 mg⋅kg-1⋅min-1; sustaining dose, 0.01 mg⋅kg-1⋅min-1) was administered in anesthetized dogs (n=11) during the following 60-min ischemia period simultaneously. At 60 min following KW3902 administration, the kidneys of the dogs were perfused again and urine flow and renal blood flow were measured continuously.
Analytical procedures
Adenosine Adenosine in the dialysate was measured according to the method developed by Miura et al[20]. The procedure is briefly described as follows: 100 µL of 1 mol/L acetate buffer (pH 4.5) and 4.5 µL of 40% cholroacetaldehyde were added. The preparation was incubated at 60 °C for 4 h to allow for the conversion of adenosine to a fluorescent 1, N6-ethenoadenosine. After the incubation, the sample was mixed with a double volume of alaminefreon reagent and was centrifuged at 1000×g for 3 min, and the upper aqueous phase was collected. For high performance liquid chromatography, a reverse-phase column (Nucleosil 7C18, 4.6 m×25 cm, Nagel) was maintained at 30 °C with a column oven (655A-52, Hitachi Ltd, Tokyo, Japan). An isocratic elution with 7.5% acetonitrile in 20 mmol/L potassium phosphate buffer (pH 5.7) at a flow rate of 1.32 mL/min was performed with a pump (L-600, Hitachi Ltd, Tokyo, Japan). Fifty microliters of the sample were injected with an autosampler (655A-40, Hitachi Ltd, Tokyo, Japan) and the elution was monitored using a fluorescence spectrometer (F-1000, Hitachi Ltd, Tokyo, Japan) at an excitation wavelength of 280 nm and an emission wavelength of 410 nm. A chromato integrator (D-2000, Hitachi Ltd, Tokyo, Japan) was used for recording.
Inosine, hypoxanthine, xanthine, and uric acid One hundred microliters of dialysate were directly applied onto the reverse-phase HPLC column (Nucleosil 7C18, 4.6 mm×25 cm, Nagel). The column was developed isocratically with 3.5% methanol in 10 mmol/L KH2PO4 at a flow rate of 1 mL/min. The effluent was monitored at a wavelength of 254 nm by an ultraviolet monitor. In every 10 samples, a standard sample was also injected.
Statistical analysis The values were presented as mean±SD. Statistical comparisons of mean values across multiple treatment groups were performed by a one-way ANOVA as appropriate, followed by Scheffe’s post hoc test. A P-value below 0.05 was taken to indicate significant difference.
Results
Effects of IPC on renal hemodynamics During 60-min renal ischemia, RBF and UF decreased from 78±18 mL/min to 0, and 260±80 µL/min to 0 respectively, whereas MBP increased slightly (Figure 1). After the release of the renal arterial clamp, RBF and UF increased to 59±27 mL/min, 170±150 µL/min, respectively (Table 1). In 1, 2, and 3 cycles of the preconditioning groups, RBF and UF descended to 0 during 60-min renal ischemia. During the reperfusion period, RBF increased to 76±11, 74±15, and 119±18 mL/min in 1, 2 and 3 cycles of the preconditioning of kidneys respectively (Table 1). UF gradually increased to 180±100, 110±70, and 260±170 µL/min in 1, 2, and 3 cycles of the preconditioning of ischemic kidneys, respectively. However, the recovery time of UF and RBF in the 3 cycles of the preconditioning of ischemic kidneys was markedly shorter compared to those of the 1 cycle or 2 cycles preconditioning of ischemic kidneys which were evaluated by percentage of changes in RBF and UF respectively (Figure 2, 3).
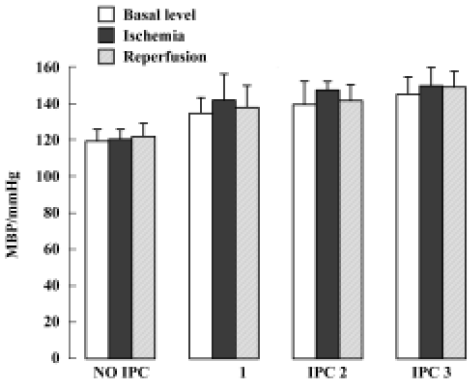
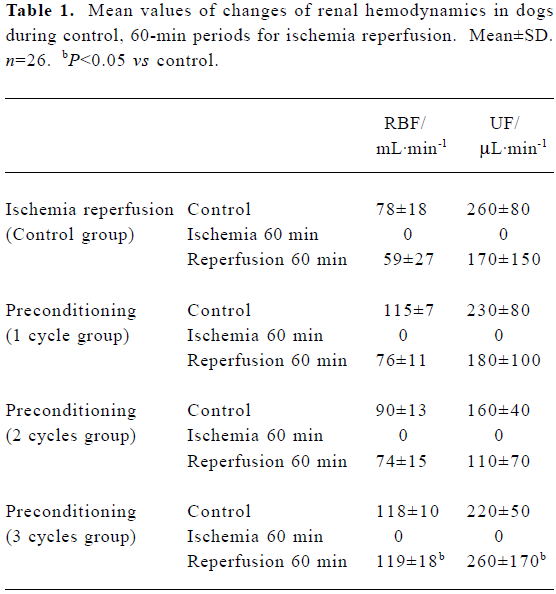
Full table
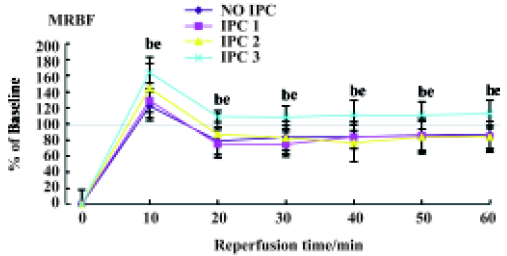
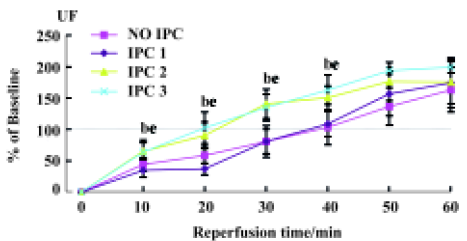
Effects of IPC on the renal interstitial concentrations of adenosine and adenine nucleotides After clamping of the renal artery, adenosine, inosine, hypoxanthine, xanthine, and uric acid concentrations increased from 0.49 to 8.21 µmol/L, 0.16 to 0.85 µmol/L, 3.61 to 10.09 µmol/L, 0.72 to 7.50 µmol/L, and 1.89 to 3.20 µmol/L, respectively. After release of the clamp, adenosine, and other nucleotides concentrations, returned to their respective pre-ischemic levels in all cases. IPC1, IPC2, and IPC3 in Figure 4 show the renal interstitial concentrations of adenosine, inosine, hypoxanthine, xanthine and uric acid during 60-min ischemia and reperfusion in response to the 1, 2, and 3 cycles of IPC, respectively. In the single cycle of the preconditioning of kidneys during ischemia, adenosine, inosine, hypoxanthine, xanthine, and uric acid levels increased to 8.04, 0.42, 7.90, 7.50, and 1.84 µmol/L, respectively. The concentration of adenosine, inosine, hypoxanthine, xanthine, and uric acid was lower than that of the non-preconditioning ischemic kidneys (adenosine, inosine, and hypoxanthine, P<0.05). Similar patterns were also observed in the 2 and 3 cycles of the preconditioning ischemic kidney. However, the levels of adenosine, inosine, hypoxanthine, xanthine, and uric acid were markedly lower in 3 cycles of the preconditioning of ischemic kidneys than those in 1 and 2 cycles of preconditioning or non- preconditioning of ischemic kidneys (hypoxanthine and xanthine, P<0.01; adenosine, P<0.05; inosine and uric acid: P>0.05. Figure 4).
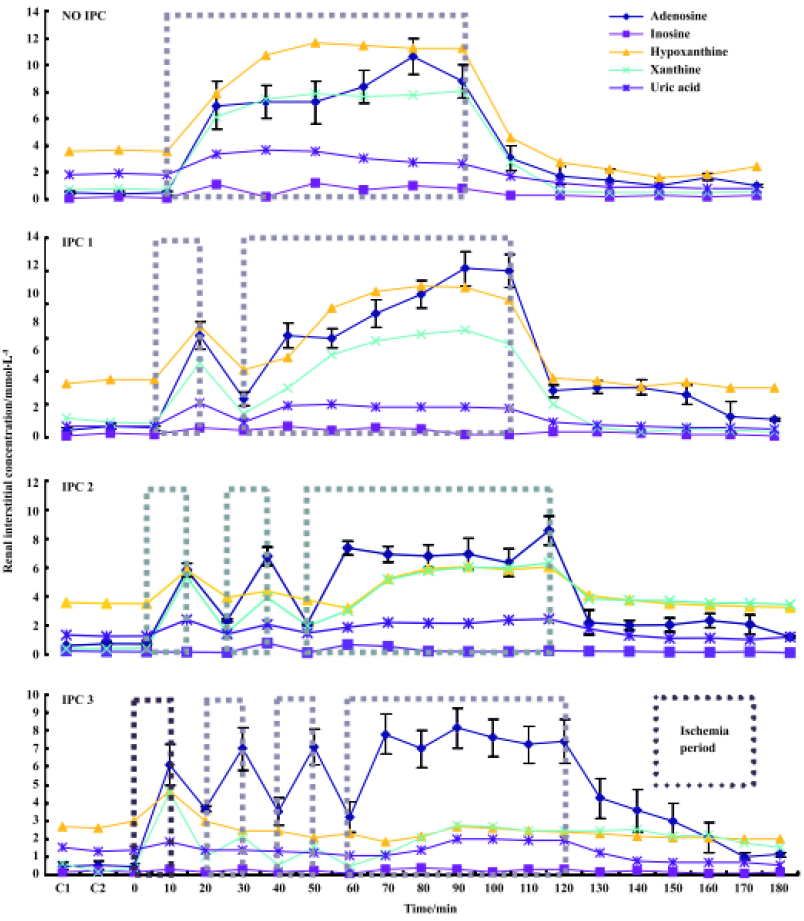
Effects of ischemia-reperfusion on renal hemodynamics during treatment with KW3902 An adenosine A1 receptor antagonist, KW3902, shortened the recovery time of renal blood flow and urine flow, respectively, compared with the control groups during the reperfusion state following 60-min renal ischemia (Figure 5).
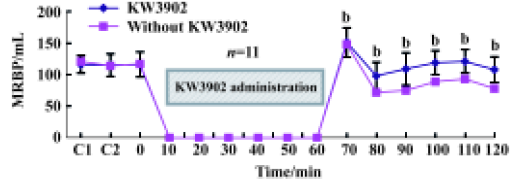
Discussion
The detrimental effects of renal ischemia-reperfusion injury, which are evident by increases in blood urea nitrogen (BUN) and creatinine (Cr)[4], reduction in the glomerular filtration rate (GFR) and renal blood flow (RBF), as well as morphological renal injury, have been well-studied in vivo or in vitro experiments. In the present study, we recognized mongrel dog renal hemodynamics parameters, urine flow (UF), RBF, mean blood pressure (BP), as well as renal interstitial adenosine and adenine nucleotide as experimental measurements using microdialysis sampling technology. Since the protective effects of ischemic preconditioning against ischemia-reperfusion injury are, at least in part, mediated by sympathetic nerves, as the protective effects were abolished by denervation[21], we denervated the dog kidneys before the experiments. Generally, during the renal 60-min ischemia followed by the 60-min reperfusion, the hemodynamic results were consistent with the data from previously published literature, returning to the respective control level quite slowly.
In 1986, it was first discovered by Murry et al[22] that multiple brief ischemia, which was defined as ischemic preconditioning (IPC) from then on, followed by the prolonged ischemia-reperfusion periods, could strengthen ischemia endurance of dog cardiac muscle and protect against post-ischemic myocardial injury and dysfunction. To date, a variety of studies have confirmed that IPC has species and organ generalization. However, evidence supporting a role for IPC in protecting against ischemia-reperfusion injury in kidneys is minimal and controversial[8,10,22-25]. In the present study, we proved that IPC with 1, 2, or 3 cycles of 10-min ischemia followed by 10-min reperfusion markedly increased 60-min post-ischemic UF and RBF, indicating the protective effects of IPC on dog renal ischemia-reperfusion injury. It is evident from our experimental results that IPC of 1 to 3 cycles with a 10-min interval shortened the recovery time of UF, RBF, and BP more significantly than that of without IPC in advance, which parallels the data reported in cardiac[6]. Previous studies[8,23] have suggested that IPC with brief cycles of ischemia and reperfusion is not effective in kidneys. However, recent studies[4,10,24,25], including the present one, have confirmed that there is an IPC effect in rat kidneys as well as dog kidneys. The discrepancies between the results of the earlier and recent studies might have arisen from the different preconditioning schedules.
A single cycle of brief ischemia is thought to be all that is needed to induce preconditioning, but most laboratories often use repetitive cycles of brief ischemia to the phenomenon. However, the beneficial effects of using multiple cycles over single cycle IPC is poorly understood. To clarify, we examined the effects of 1, 2, or 3 cycles of IPC on the renal function in ischemia-reperfusion kidney. As shown in our study, along with the increase of cycles of 10-min IPC, less recovery time during the 60-min reperfusion following 60-min ischemia is needed. To our knowledge, this is the first study that determines the cycles of 10-min renal IPC and the recovery time of BP, UF as well as RBF during 60-min renal reperfusion following 60-min renal ischemia, respectively, in dogs. The post-ischemic recovery of BP, UF as well as RBF occurred faster than that without IPC, and recovery time of BP, UF as well as RBF inversely related to the number of cycles, that is, more cycles of 10-min IPC (within 3 cycles), the shorter recovery time of BP, UF as well as RBF during the 60-min renal reperfusion following 60-min renal ischemia, respectively. Collectively, we can speculate that more cycles of renal IPC can protect against the renal ischemia-reperfusion injury better. In our present study, 10-min ischemia time intervals were chosen, which performed well during the experiment, even though it had been reported that 10-min renal ischemia produced some degree of cellular injury[8]. In our future experiments, we will increase the cycles of IPC and change the ischemia intervals. We will also prolong monitoring time after 60-min renal ischemia following different cycles of IPC in order to investigate the optimum duration of IPC and whether the “memory function” exists in the effect of IPC, that is, the protective effect of renal ischemic preconditioning under “optimum duration of IPC” can last very long time.
The precise mechanism of ischemic preconditioning, even in cardiac muscle, is not fully elucidated. However, numerous studies have been carried out to elucidate the mechanism of IPC, and special attention has been given to the finding that adenosine pretreatment mimics ischemic preconditioning in cardiac and renal tissue, and adenosine appears to be mediator of ischemic preconditioning[6,26,27]. Adenosine is released in large quantities within seconds after the onset of ischemia, including in the kidneys. The general concept is that a build-up of endogenous adenosine during the brief ischemia period (IPC period) can trigger protection via activation of adenosine receptors.
Previous studies suggest that renal ischemia increases the renal interstitial concentrations of adenosine and adenine nucleotide[11], and increased extracellular adenosine has been suggested as a regulator of renal hemodynamics in postischemia[15–17]. However, these findings are indirect and do not provide any data about the renal interstitial concentrations of adenosine and adenine nucleotides during IPC followed by ischemia and reperfusion. Therefore, the present study was performed to determine the levels of adenosine and adenine nucleotide in renal interstitial fluid during preconditioning followed by ischemia and reperfusion. Our results show that levels of adenosine and adenine nucleotides increase during IPC and the ischemic period. However, during ischemia, levels of adenosine and adenine nucleotides in renal interstitial fluid were lower than those of non-IPC kidney, and among the IPC groups, renal interstitial concentrations of adenosine and adenine nucleotides were markedly lower in ischemic kidney of 3 cycles IPC groups. The mechanism of IPC that decreased the levels of adenosine and adenine nucleotide during the ischemic period is not clear. Further research is necessary to evaluate how IPC lower renal interstitial concentrations of adenosine and adenine nucleotide during ischemic period.
Previous studies have shown that IPC improves the post-ischemic recovery of UF in rats[10]. In that study, the rats were subjected to 2 cycles of 3-min ischemia and 5-min reperfusion. The UF was examined at 90–120 min after ischemia. In the present experiment, dogs were subjected to 1, 2, or 3 cycles of 10-min ischemia and 10-min reperfusion. Our results also show that IPC improves the post-ischemic recovery of UF within 60 min. However, one important finding of our study is that IPC with 3 cycles is more protective compared to single or 2 cycles of IPC. The mechanism of more protective effects of IPC with multiple cycles is not clear. However, our results show that renal interstitial concentrations of adenosine and nucleotides are inversely related to the number of cycles. Therefore one possibility is that renal protective effect of IPC with 3 cycles is more effective because of lower renal interstitial concentrations of adenosine and adenosine nucleotides during ischemic insult.
At present, only 2 studies using the long duration of multiple cycles IPC have suggested the role of adenosine in IPC in the rat kidney[4,10]. In those studies, they have demonstrated that adenosine pretreatment or adenosine A1 receptor activation by adenosine A1 receptor agonist before ischemic insult protects post-ischemic renal function. However, Sugino et al[10] reported that adenosine A1 receptor antagonist failed to abolish the post-ischemic renal protective effects of IPC or adenosine infusion in the rat kidney. In our study, the results using adenosine A1 receptor antagonist consequently seem to indicate that the adenosine A1 receptor activation deteriorates the renal function, supporting previously published studies[28], but is contradictory to the finding of Lee HT[4]. Agmon Y et al[29] found that interrenal adenosine reduced cortical blood flow and predominantly increased medullary flow via the A1 and A2 receptors, respectively. Also, it has been suggested[30] that vasoconstriction following brief renal artery occlusion is mediated by an increased accumulation of adenosine during the period of ischemia. Nishiyama et al[31], however, suggested that ischemia-induced accumulation of renal interstitial adenosine elicited renal vasoconstriction, at least in part, but might not have been the primary mediator of renal vasoconstriction in the maintenance phase of postischemia. At present, it is also suggested that renal IPC initiates the protective endocellular system and kidneys can release several kinds of interstitial mediators mediating the conduct of cell signals, including adenosine, which is recognized as an important factor in the protective effect of IPC. Collectively, we speculate that the conclusive effect of endogenous adenosine during ischemia-reperfusion is detrimental to the renal hemodynamics. This is because of the marked regional heterogeneity of the 3 adenosine receptor subtype distribution in dog kidneys which attenuate and prolong the recovery time of RBF and UF, respectively.
In summary, these results indicate that IPC protects post-ischemic renal function, and decreases the renal interstitial concentrations of adenosine and adenine nucleotides in ischemic kidneys. The present study showed that the shortened recovery time of BP, UF as well as RBF during the 60-min renal reperfusion following 60-min renal ischemia was progressively greater with multiple cycles of brief IPC. We have also confirmed that renal IPC can protect against ischemia-reperfusion injury. The predominant effect of endogenous adenosine induced by renal ischemia is detrimental to renal function because of the marked regional heterogeneity of the 3 adenosine receptor subtype distribution in dog kidneys.
Acknowledgements
We are grateful to Hidehiko SAKURAI and Motoki KYO (Toyobo, Otsu, Japan) for supplying the dialysis membrane and steel needles. Our thanks also to Kyowa Hakko Kogyo Co Ltd, for supplying KW-3902, and to Yumi IHARA for secretarial service.
References
- Kerrigan CL, Stotland MA. Ischemia reperfusion injury: a review. Microsurgery 1993;14:165-75.
- Aronson S, Blumenthal R. Perioperative renal dysfunction and cardiovascular anesthesia: concerns and controversies. J Cardio-thorac Vasc Anesth 1998;12:567-86.
- Huet F, Gouyou JB, Guignard JP. Prevention of hypoxemia-induced renal dysfunction by perindoprilat in the rabbit. Life Sci 1997;61:2157-65.
- Lee HT, Emala CW. Protective effects of renal ischemic preconditioning and adenosine pretreatment: role of A1 and A3 receptors. Am J Physiol Renal Physiol 2000;278:F380-7.
- Nishiyama A, Miyatake A, Aki Y, Fukui T, Rahman M, Kimura S, et al. Adenosine A1 receptor antagonist KW-3902 prevents hypoxia-induced renal vasoconstriction. J Pharmacol Exp Ther 1999;291:988-93.
- Meldrum DR. Mechanisms of cardiac preconditioning: ten years after the discovery of ischemic preconditioning. J Surg Res 1997;73:1-13.
- Reimer KA, Jennings RB. Ischemic preconditioning: a brief review. Basic Res Cardiol 1996;91:1-4.
- Islam CF, Mathie RT, Dinneen MD, Kiely EA, Peters AM, Grace PA. Ischaemia-reperfusion injury in the rat kidney: the effect of preconditioning. Br J Urol 1997;79:842-7.
- Turman MA, Bates CM. Susceptibility of human proximal tubular cells to hypoxia: effect of hypoxic preconditioning and comparison to glomerular cells. Ren Fail 1997;19:47-60.
- Sugino H, Shimada H, Tsuchimoto K. Role of adenosine in renal protection induced by a brief episode of ischemic preconditioning in rats. Jpn J Pharmacol 2001;87:134-42.
- Nishiyama A, Kimura S, He H, Miura K, Rahman M, Fujisawa Y, et al. Renal interstitial adenosine metabolism during ischemia in dogs. Am J Physiol Renal Physiol 2001;280:F231-8.
- Navar LG, Inscho EW, Majid SA, Imig JD, Harrison-Bernard LM, Mitchell KD. Paracrine regulation of the renal microcircula-tion. Physiol Rev 1996;76:425-536.
- Spielman WS, Arend LJ. Adenosine receptors and signaling in the kidney. Hypertension 1991;17:117-30.
- Spielman WS, Thompson CI. A proposed role for adenosine in the regulation of renal hemodynamics and renin release. Am J Physiol 1982;242:F423-35.
- Lin JJ, Churchill PC, Bidani AK. Effect of theophylline on the initiation phase of postischemic acute renal failure in rats. J Lab Clin Med 1986;108:150-4.
- Lin JJ, Churchill PC, Bidani AK. The effect of dipyridamole on the initiation phase of postischemic acute renal failure in rats. Can J Physiol Pharmacol 1987;65:1491-5.
- Lin JJ, Churchill PC, Bidani AK. Theophylline in rats during maintenance phase of post-ischemic acute renal failure. Kidney Int 1988;33:24-8.
- Rahman M, Kimura S, Yoneyama H, Kasaka H, Nishiyama A, Fukui T, et al. Effects of furosemide on the tubular reabsorption of nitrates in anesthetized dogs. Eur J Pharmacol 2001;428:113-9.
- Nishiyama A, Miyatake A, Kusudo K, Syokoji T, Yue W, Fukui T, et al. Effects of halothane on renal hemodynamics and interstitial nitric oxide in rabbits. Eur J Pharmacol 1999;367:299-306.
- Miura K, Okumura M, Yukimura T, Yamamoto K. Fluorometric determination of plasma adenosine concentrations using high-performance liquid chromatography. Anal Biochem 1991;196:84-8.
- Ogawa T, Mimura Y, Kaminishi M. Renal denervation abolishes the protective effects of ischaemic preconditioning on function and haemodynamics in ischaemia-reperfused rat kidneys. Acta Physiol Scand 2002;174:291-7.
- Murry CE, Jennings RB, Reimer KA. Preconditioning with ischemia: A delay of lethal cell injury in ischemic myocardium. Circulation 1986;74:1124-36.
- Zager RA, Jurkowitz MS, Merola AJ. Responses of the normal rat kidney to sequential ischemic events. Am J Physiol 1985;249:F148-59.
- Riera M, Herrero J, Torras J, Cruzado JM, Fatjo M, Lloberas N, et al. Ischemic preconditioning improves postischemic acute renal failure. Transplant Proc 1999;31:2346-7.
- Cochrane J, Williams BT, Banerjee A, Harken AH, Burke TJ, Cairns CB, et al. Ischemic preconditioning attenuates functional, metabolic, and morphologic injury from ischemic acute renal failure in the rat. Ren Fail 1999;21:135-45.
- Liu GS, Thornton J, Van Winkle DM, Stanley AW, Olsson RA, Downey JM. Protection against infarction afforded by preconditioning is mediated by A1 adenosine receptors in rabbit heart. Circulation 1991;84:350-6.
- Thornton JD, Liu GS, Olsson RA, Downey JM. Intravenous pretreatment with A1-selective adenosine analogues protects the heart against infarction. Circulation 1992;85:659-65.
- Rossi N, Ellis V, Kontry T, Gunther S, Churchill P, Bidani A. The role of adenosine in HgCl2-induced acute renal failure in rats. Am J Physiol 1990;258:F1554-60.
- Agmon Y, Dinour D, Brezis M. Disparate effects of adenosine A1- and A2-receptor agonists on intrarenal blood flow. Am J Physiol 1993;265:F802-6.
- Spielman WS, Osswald H. Blockade of postocclusive renal vasoconstriction by an angiotensin II antagonists: evidence for an angiotensin-adenosine interaction. Am J Physiol 1979;237:F463-7.
- Nishiyama A, Miura K, Miyatake A, Fujisawa Y, Yue W, Fukui T, et al. Renal interstitial concentration of adenosine during endotoxin shock. Eur J Pharmacol 1999;385:209-16.