Action of aluminum on high voltage-dependent calcium current and its modulation by ginkgolide B
Introduction
The accumulation of aluminum (Al) within the body can result in many mental diseases. For example, Al is concentrated in the neurofibrillary tangles and senile plaques of patients with Alzheimer disease (AD)[1]. Al can be attributed to several neurological disorders, such as dialysis syndrome and Guamanian amylotrophic lateral sclerosis-Parkinson’s dementia[2]. A number of studies have implicated that Al has no effect on long-term potentiation (LTP)[3]. However, more and more studies have shown that Al can impair LTP[4] and evoke potential in the hippocampus[5]. Studies have also shown that Al affects amino acid neurotransmitters in the hippocampus and enhances glutamate-mediated excitotoxi-city, which may be one of the causes of its toxicity[6]. Reports about its mechanism involving ion channels are few and controversial[7–9].
Extracts from leaves of Ginkgo biloba (EGb) and one of its constituents ginkgolide B have been demonstrated to protect cardiomyocytes and cultured neurons from the injury induced by hypoxia, ischemia, and the neurotoxicity induced by Aβ[10–12]. However, it is not known whether the mechanism of this protection of neurons involves ion channels, such as voltage-dependent calcium channels (VDCC).
The present study investigated the actions of Al on IHVA and its modulation by Gin B to examine the neurotoxic mechanisms of Al and the neuroprotective mechanisms of Gin B.
Materials and methods
Reagents Pronase E, forskolin, TEA-Cl, H-89, and HEPES were purchased from Sigma Chemical Company (St Louis, MO, USA). H-89 was dissolved in pipette solution and stored at -20°C. After the whole-cell configuration was constructed, H-89 was dialyzed into the cell through the pipette. Ginkgolide B (BN52021, purity 98.2%) was from the Wuhan Institute of Botany, Chinese Academy of Sciences (Wuhan, China). AlCl3 was from Jinghua Chemical Company (Beijing, China). The remaining chemicals, unless otherwise stated, were all purchased from the Shanghai Chemical Reagent Plant (Shanghai, China).
Cell isolation Animals were provided by the experimental animal center of Tongji Medical College (Grade II, Certificate N
Electrophysiology The cells were placed in a recording chamber mounted on the stage of an inverted microscope (Carl Zeiss, Germany) and superfused with extra cellular solution at room temperature (21–22 °C). Extracellular solution for recording IHVA was composed of (mmol/L): NaCl 150, KCl 5, MgCl2 1.1, CaCl2 2.5, HEPES 10, glucose 10, TTX 0.001, and the pH was adjusted to 7.4 with NaOH. Extracellular application of drugs was carried out by perfusing cells with extracellular solution containing the drugs.
Whole-cell patch experiments were carried out using an Axopatch 200A amplifier (Axon Instruments, Foster City, CA, USA) driven by ISO2 software (MFK, Frankfurt, Germany). In the voltage-clamp experiments, the cells were stepped from -80 mV (50 ms) to -40 mV (200 ms), and then depolarized to 0 mV (200 ms) after briefly hyperpolarizing the membrane potential for 10 ms to -45 mV. The IHVA was activated by the second depolarization. The protocol was applied every 5 s. For analysis of the current-voltage (I–V) relationship, voltage steps (200 ms) were used to depolarize from -40 mV to +40 mV in 10 mV increments. Glass pipettes were used with a resistance of about 3–5 MΩ when filled with a pipette solution composed of (mmol/L): CsCl 140, MgCl2 2, Mg-ATP 4, TEA-Cl 2, HEPES 10, egtazic acid 11, and the pH was adjusted to 7.2 with CsOH.
Data were acquired at a sampling rate of 10 kHz, filtered at 2 kHz, stored on hard disk and analyzed off-line using the ISO2 analysis software package (MFK, Frankfurt, Germany).
Data analysis The amplitude of IHVA was calculated as the difference between the instantaneous current at the beginning of the experiment and the maximum activating current. Currents were normalized to membrane capacitance to calculate current densities (pA·pF-1). Cell membrane capacitance (Cm) was determined online using the ISO2 software program. The activation rate constant and inactivation rate constant were obtained using the ISO2 analysis software. Graphical and statistical data analyses were carried out using Sigmaplot 2001 (SPSS, Chicago, IL, USA) and Origin 6.0 (Microcal Software, Inc, Northampton, MA01060, USA). Data were presented as mean±SEM where appropriate. Statistical analysis were carried out using Student’s paired and unpaired t-tests and values of P<0.05 were considered statistically significant.
Results
Action of Al on IHVA Bath application of AlCl3 0.01 mmol/L had no effect on IHVA. The current densities before and after AlCl3 application were 18.5±2.4 pA·pF-1 and 18.5±2.2 pA·pF-1, respectively (n=11, P>0.05) (Figure 1A).
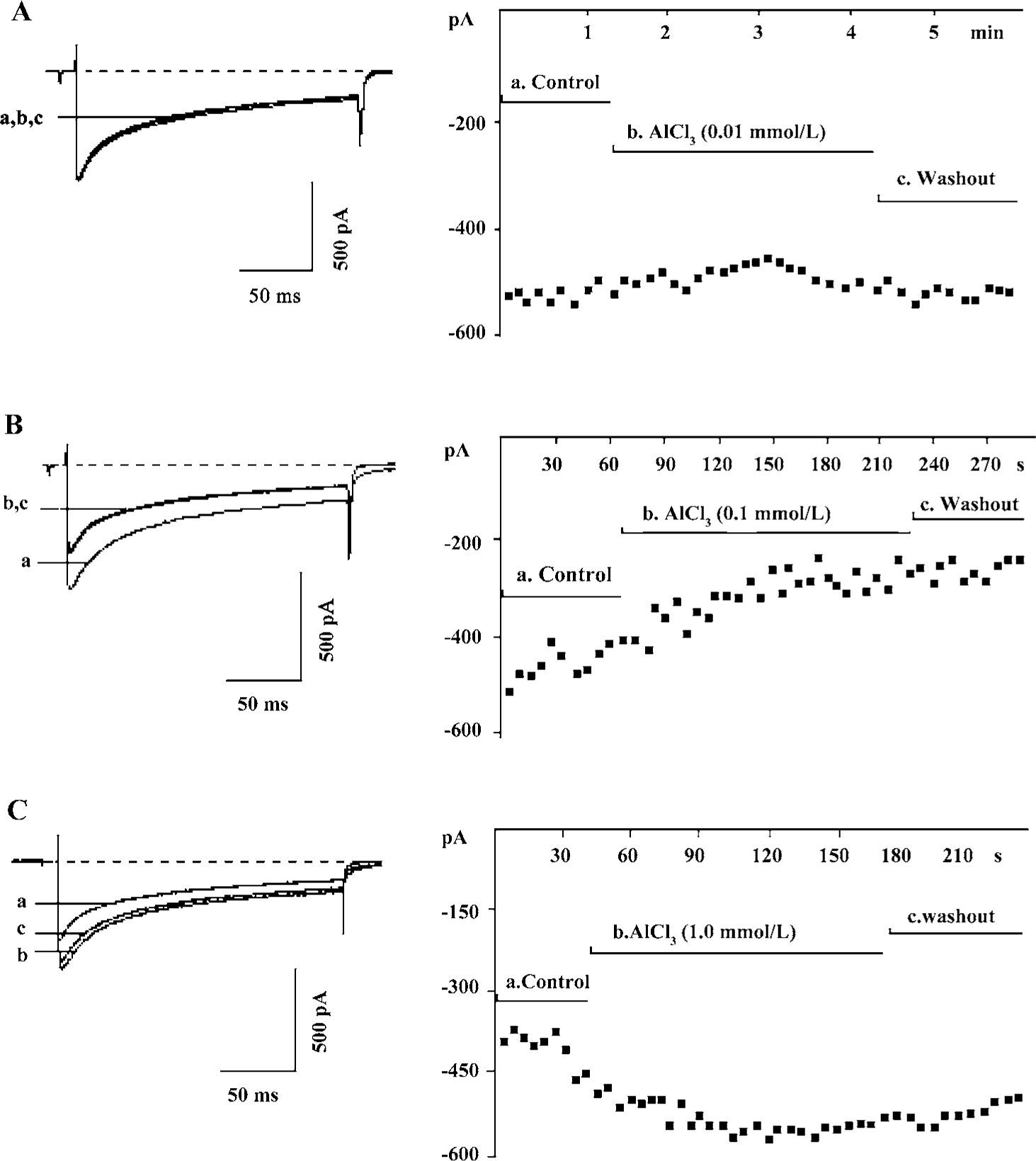
Bath application of AlCl3 0.1 mmol/L caused a reduction in IHVA from 17.7±1.6 pA·pF-1 to 12.7±1.4 pA·pF-1 (n=27, P<0.01), that is, a reduction of 30.5%± 4.1%. The reduction of IHVA by AlCl3 did not recover after the AlCl3 was washed out (Figure 1B).
AlCl3 0.25 mmol/L caused a reduction in IHVA in 80% (8/15) of the neurons, and an increase in 20% (4/15) of the neurons. AlCl3 0.50 mmol/L caused a reduction in IHVA in 50% (7/14) of the neurons, and an increase in IHVA in 50% (7/14) of the neurons. In contrast, AlCl3 0.75 mmol/L increased IHVA by 30.8%±5.2% (n=15, P<0.01) in all neurons tested (from 17.8±1.8 pA·pF-1 to 23.0±2.5 pA·pF-1). AlCl3 1.0 mmol/L increased IHVA by 37.3%±7.8% (from 19.6±3.1 pA·pF-1 to 26.2±4.3 pA·pF-1) (n=21, P<0.01). IHVA increased by AlCl3 was irreversible after AlCl3 was washed out (Figure 1C).
At both low and high concentrations, AlCl3 inhibited or increased the maximum amplitude of IHVA, but had no effect on the activation threshold potential of IHVA in the I–V relationship (Figure 2A, B). The G-V curve was unaffected by AlCl3 0.1 mmol/L (n=5, P>0.05) or AlCl3 1.0 mmol/L (n=5, P>0.05) (control: V0.5=-12.8 mV±4.4 mV, k=5.5±3.8; AlCl3 0.1 mmol/L: V0.5=-12.0 mV±4.5 mV, k=5.0±4.0; AlCl 3 1.0 mmol/L: V0.5=-13.6 mV±5.3 mV, k=5.2±4.4) (Figure 2C). In addition, AlCl3 had no effect on the activation rate constants at concentrations of 0.1 mmol/L (n=8, P>0.05) or 1.0 mmol/L (n=9, P>0.05).
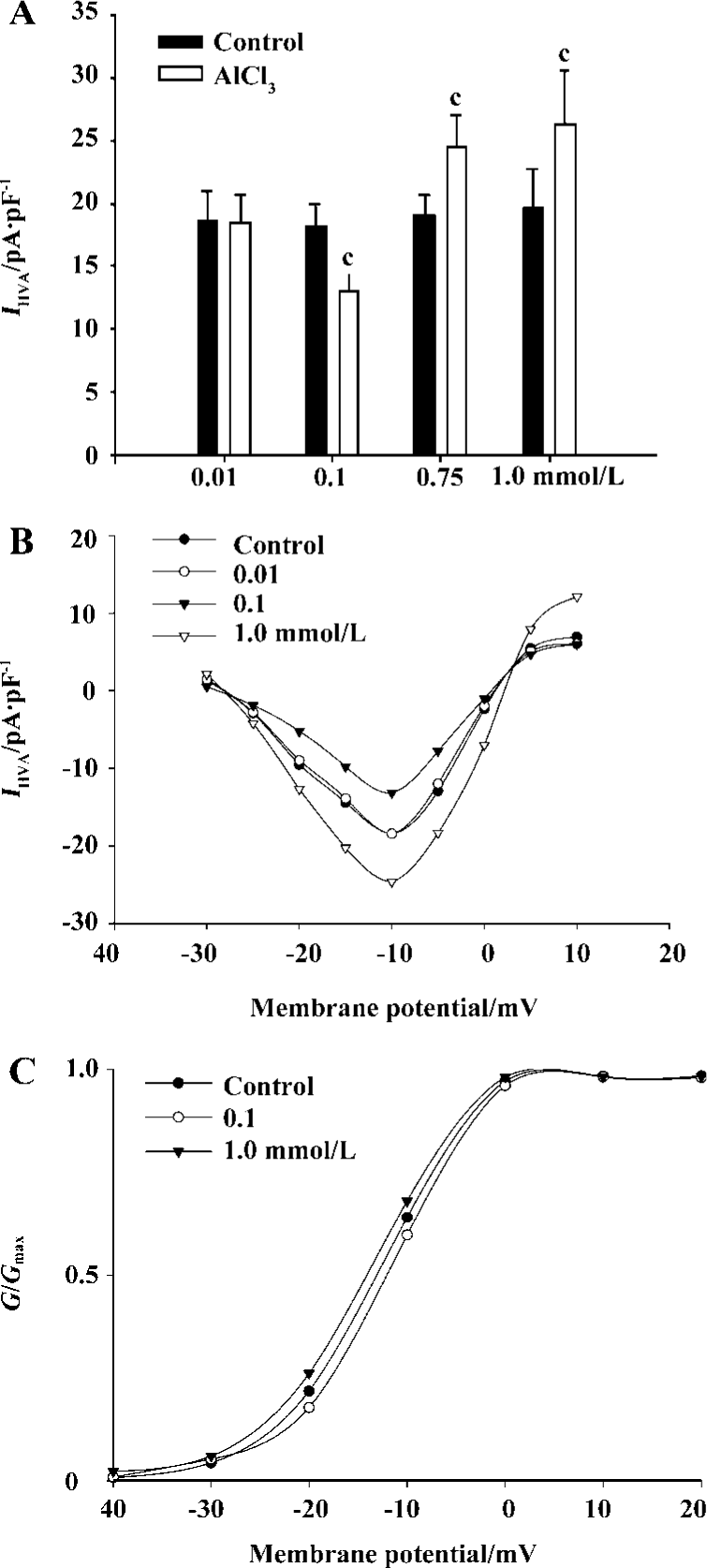
To gain a better understanding of the action of Al on IHVA, we explored its action on the steady-state inactivation curve of IHVA. AlCl3 shifted the curve to a depolarizing voltage at 1.0 mmol/L (n=5, P<0.05), whereas it shifted the inactivation curve to a hyperpolarizing voltage at 0.1 mmol/L (n=5, P<0.05). (Control: V0.5=-35.4±3.3 mV, k=-14.3±2.5; 0.1 mmol/L AlCl3: V0.5=-41.1±2.7 mV, k=-9.2±2.0; 1.0 mmol/L AlCl3: V0.5=-29.8±6.9 mV, k=-10.8±2.4). AlCl3 0.1 mmol/L decreased the inactivation rate constant by 27.3%±6.3% (n=5, P<0.01), whereas 1.0 mmol/L AlCl3 increased the inactivation rate constant by 44.7%±3.4% (n=7, P<0.01) (Figure 3).
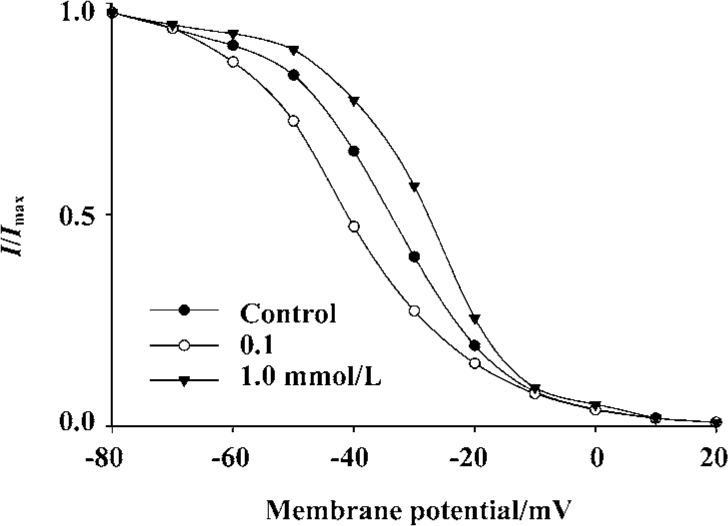
Effect of Gin B on IHVA in hippocampal neurons Gin B at doses of 0.01–20 µmol/L had no effect on IHVA in normal hippocampal neurons (P>0.05) (Table 1). Gin B inhibited the increase of IHVA by AlCl3 1.0 mmol/L. After a steady increase in the action of AlCl3, Gin B at concentrations of 0.01 µmol/L, 0.1µmol/L, 1.0 µmol/L, and 10 µmol/L reduced IHVA by 21.0%±4.6% (n=7, P<0.05), 57.9%±7.8% (n=6, P<0.01), 79.3%±2.7% (n=6, P<0.01), and 82.4%±7.3% (n=6, P<0.05), respectively. The concentration producing 50% inhibition by Gin B of Al 1.0 mmol/L is 0.0359 µmol/L±0.0038 µmol/L (Figure 4).
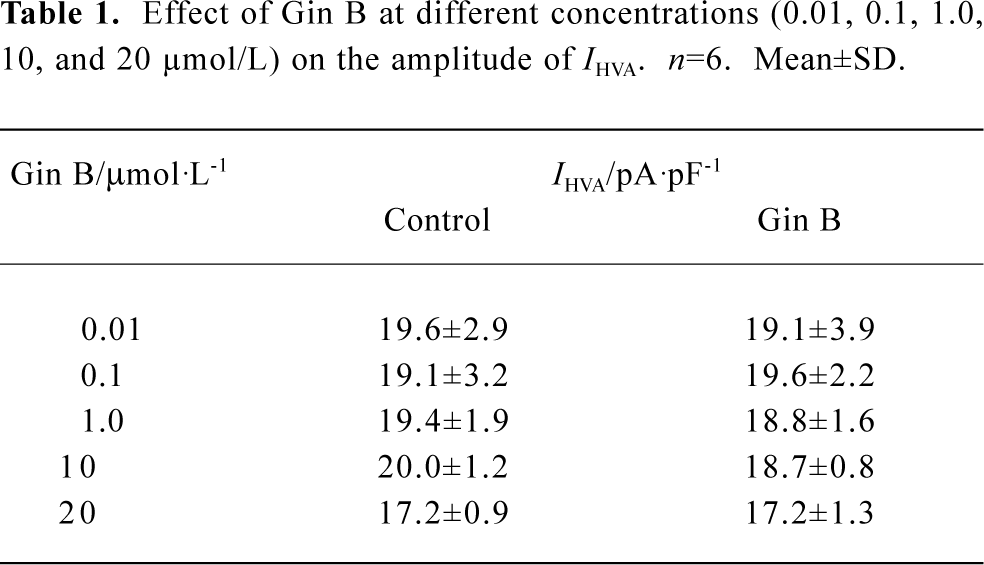
Full table
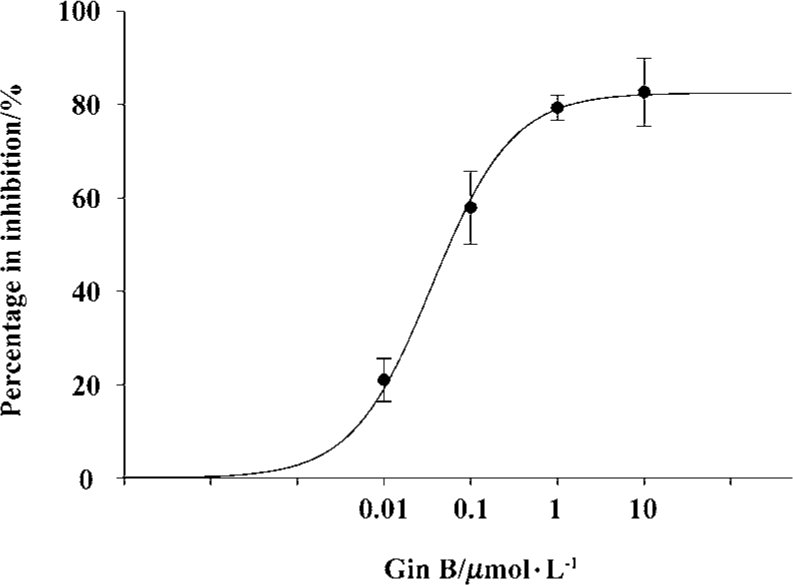
Co-superfusion AlCl3 0.1 mmol/L plus Gin B 10 µmol/L was applied in the same way as AlCl3 1.0 mmol/L. For all tested neurons (n=15), there was no change in IHVA in 53.3% of the neurons and a slight increase in IHVA in the remaining neurons (P>0.05). This result indicated that Gin B had no effect on the action of Al 0.1 mmol/L .
Mechanism of action of high concentrations of Al on IHVA Application of forskolin 10 µmol/L (an agonist of adenylyl cyclase) increased IHVA by 30.8%±7.5% (n=14, P<0.05). Bath application of forskolin 10 µmol/L in combination with Al 1.0 mmol/L increased IHVA by 68.3%±8.7% (n=31, P<0.05) (Figure 5A).
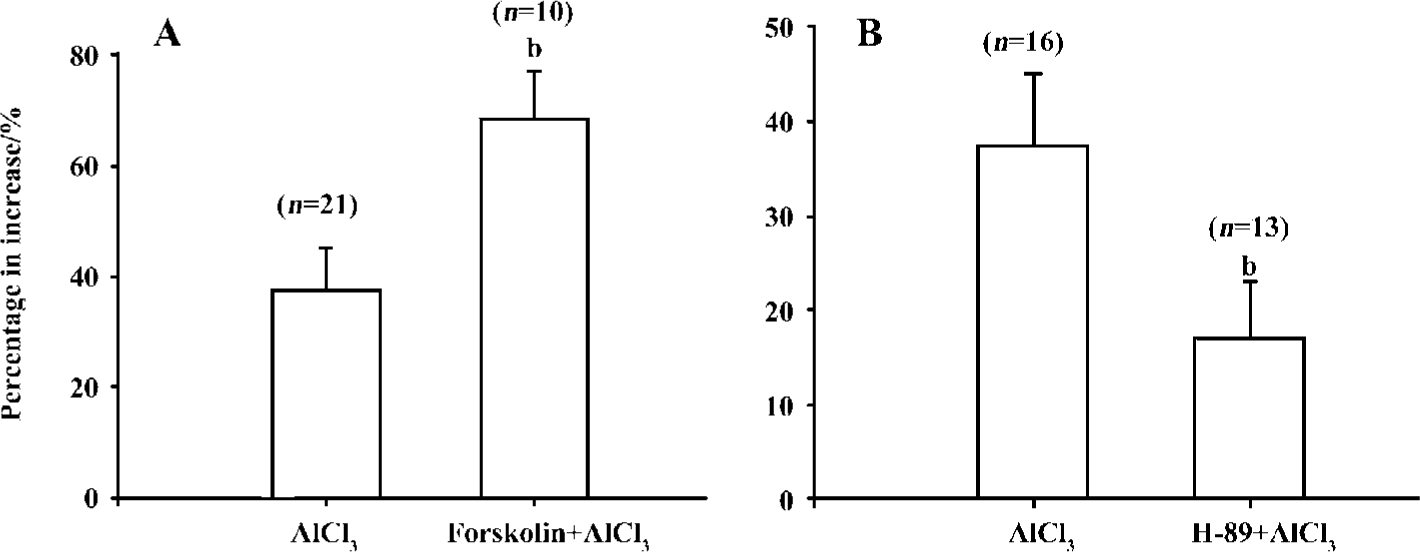
H-89 is a selective antagonist of PKA. In this study, adding H-89 in the pipette solution reduced the amplitude of IHVA by 42.0%±4.1% (from 20.2±3.3 pA·pF-1 to 13.9±3.1 pA·pF-1, n=6, P<0.01) within approximately 80–100 s. AlCl31.0 mmol/L was bath applied in the presence of H-89 (10 µmol/L) in the pipette solution. Aluminum increased IHVA by 17.2%±5.8% (n=10, P<0.05). Compared with the effect of Al 1.0 mmol/L on IHVA without H-89 (n=29, P<0.05), the reduction in IHVA in the presence of H-89 was significant, indicating that H-89 could, in part, abolish the increase in IHVA by Al at high concentrations (Figure 5B).
Mechanism of action of low concentrations of Al on IHVA To investigate the mechanism by which Al inhibited IHVA at low concentrations, AlCl3 0.1 mmol/L was applied first and IHVA was reduced to 12.9±1.1 pA·pF-1 from 18.5±1.7 pA·pF-1 (n=12, P<0.01). After the current was stable, fors-kolin 10 µmol/L and AlCl3 0.1 mmol/L were co-applied. Fors-kolin did not cancel the inhibition of IHVA by 0.1 mmol/L AlCl3. The IHVA after forskolin application was 12.5±0.9 pA·pF-1 (n=12, P>0.05) (Figure 6A).
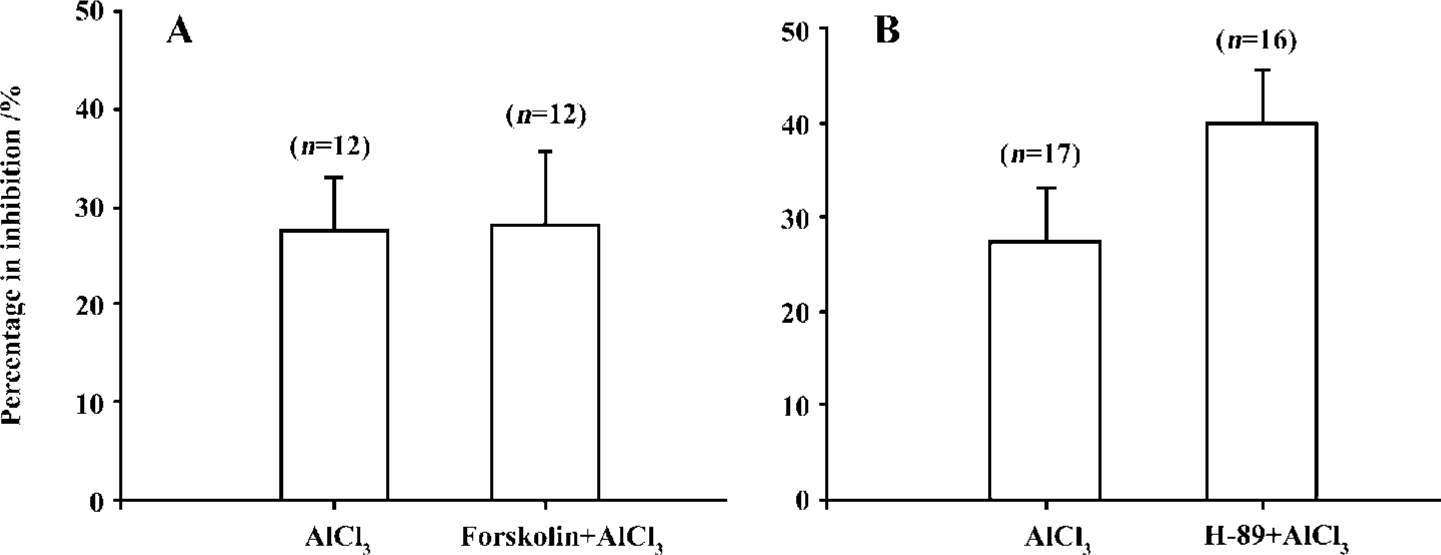
In the presence of H-89 (10 μmol/L), IHVA was reduced to 13.1±2.5 pA·pF-1 from 20.1±4.2 pA·pF-1 (n=8, P<0.01). After the current was stable, AlCl3 0.1 mmol/L was bath applied, and IHVA was reduced to 12.5±2.5 pA·pF-1. There was no difference in the percentage inhibition with and without H-89 application (n=33, P>0.05) (Figure 6B).
Discussion
VDCC in hippocampal neurons are divided into high voltage-dependent channels (HVA) and low voltage-dependent channels (LVA) according to the difference in activation threshold. In the present study we demonstrated that the effect of Al on IHVA differed at different concentrations. Al reduced the amplitude of IHVA irreversibly at low concentrations (0.1 mmol/L). This result supports a previous report on dorsal root ganglion (DRG) neurons[7]. However, Al inhibited and enhanced IHVA as Al concentrations increased (between 0.25 mmol/L and 0.50 mmol/L), and the percentage of enhanced IHVA by Al in the neurons examined increased with increased Al concentrations. When 0.75 mmol/L and 1.0 mmol/L Al were bath applied, the amplitude of IHVA in all neurons tested increased.
The toxic effect of Al in humans is chronic and accumulative and leads to degradation and apoptosis of cells[14]. Acute application of Al inhibits LTP on hippocampal slices of rats as well as in vivo by intracerebroventricular injection.
In our study, the actions of Al on IHVA differed at different concentrations; thus, it is possible that the mechanism of action is different at different Al concentrations. Protein phosphorylation modulates the function of VDCC and the AC-cAMP-PKA system plays a key role[17]. Thus, forskolin and H-89 were used to investigate whether the action of Al on IHVA is involved in this mechanism. H-89 markedly abolished the increase of IHVA by Al 1.0 mmol/L. Co-superfusion with forskolin plus Al at high concentrations caused more Ca2+ influx. Together these results indicate that an Al-induced increase in IHVA possibly results from activating cAMP-PKA. However, H-89 did not reverse the action of AlCl3 totally, suggesting that other mechanisms must contribute to its action on IHVA at high concentrations.
Platt[7] reported that the interactions of aluminum with two different binding sites (within and outside) of calcium channels might contribute to the reduction of VDCCs on DRG neurons. Al has been reported to inhibit Mg-dependent enzymes and to interact with phosphorylation sites[18]. In the present study, the co-application of forskolin and Al did not cancel the reduction and the action of 0.1 mmol/L Al was not affected by H-89, indicating that the mechanism by which Al reduces IHVA at low concentrations might not be involved in the cAMP-PKA system. In addition, Gin B effectively canceled the increase of IHVA by Al at high con-centrations, but had almost no effect on the reduction of IHVA by Al at low concentrations, further suggesting that the action of Al at low concentrations on IHVA occurs via a different mechanism. The mechanism by which Al reduced IHVA requires further examination. At intermediate concentration ranges, Al both reduced and enhanced IHVA. The mechanism is not known, but may result from a difference in neurons or from the concentration of Al itself, which indicated that this concentration might be the point at which IHVA moves from being inhibited to enhanced and this might be the reason for its complexity and diversity.
EGb is a complex mixture containing 24% flavonoid glycosides, 6% terpene lactones, such as ginkgolide A, B, C, J and bilobalide, a number of organic acids, and various other constituents. Studies have shown that Gin B has many pharmacological effects (ie preventing atherosclerosis, diminishing coagulation of platelets, ameliorating the circulation system) and has a distinctively protective effect on the central nerve and cardiovascular systems. Clinical studies have shown that oral administration of EGb in human patients with dementia is effective[19]. Gin B can protect cardio-cmyocytes and cultured neurons from injury by hypoxia and ischemia through many pathways, for example, by acting as an anti-oxidant[20], acting as the antagonist of platelet-activating factor[21] and by inhibiting NO-stimulated PKC activity[22]. Furthermore, Gin B has been shown to prevent neurons from glutamate excitoxicity through a reduction in [Ca2+]i[23] and to have an effect on the glycine-gated chloride channel[24]. The present study provides the first evidence that Gin B can cancel the increase of IHVA by Al, and that Gin B can protect neurons by inhibiting IHVA, providing a possible mechanism for clinical treatment in a number of nervous system diseases. The detailed mechanism by which Gin B inhibits IHVA remains to be investigated.
References
- Tokutake S, Nagase H, Morisaki S, Oyanagi S. Aluminium detected in senile plaques and neurofibrillary tangles is contained in lipofuscin granules with silicon, probably as aluminosilicate. Neurosci Lett 1995;185:99-102.
- Wisniewski HM. Aluminum, tau protein, and Alzheimer’s disease. Lancet 1994;344:204-5.
- Gilbert ME, Shafer TJ. In vitro exposure to aluminum does not alter long-term potentiation or glutamate release in rat hippocampal slices. Neurotoxicol Teratol 1996;18:175-80.
- Platt B, Carpenter DO, Busselberg D, Reymann KG, Riedel G. Aluminum impairs hippocampal long-term potentiation in rats in vitro and in vivo. Exp Neurol 1995;134:73-86.
- Zou BD, Xiao HM, Zhang ZD, Li A. Effect of aluminum on evoked potential on hippocampal CA3 area in rats. J Tongji Med Univ 1998;27:15-18.
- Matyja E. Aluminum enhances glutamate-mediated neurotoxicity in organotypic cultures of rat hippocampus. Folia Neuropathol 2000;38:47-53.
- Platt B, Büsselberg D. Actions of aluminium on voltage-activated calcium channel currents. Cell Mol Neurobiol 1994;14:819-29.
- Trombley PQ. Selective modulation of GABAA receptors by aluminum. J Neurophysiol 1998;80:755-61.
- Platt B, Haas H, Büsselberg D. Aluminum reduces glutamate-activated currents of rat hippocampal neurons. Neuroreport 1994;5:2329-32.
- Zhang ZX, Qi XY, Xu YQ. Effect of ginkgolide B on L-type calcium current and cytosolic [Ca2+]i in guinea pig ischemic ventricular myocytes. Acta Physiol Sin 2003;55:24-8.
- Bastianetto S, Quirion R. EGb 761 is a neuroprotective agent against beta-amyloid toxicity. Cell Mol Biol 2002;48:693-7.
- Wu XF, Wang QJ, Lou FC. Protective effect of Ginkgolides on rat focal brain ischemia. J China Pharm Univ 2001;32:141-5.
- Xu CQ, Zhang ZM, Xia ZL, Wu CH, Zhou PA. Acute isolation and identification of CA1 pyramidal neurons in hippocampus of rats. Chin J Appl Physiol 1999;15:189-91.
- Savory J, Herman MM, Ghribi O. Intracellular mechanisms underlying aluminum-induced apoptosis in rabbit brain. J Inorg Biochem 2003;97:151-4.
- Deisseroth K, Heist EK, Tsien RW. Translocation of calmodulin to the nucleus supports CREB phosphorylation in hippocampal neurons. Nature 1998;392:198-202.
- Morgan SL, Teyler TJ. VDCCs and NMDARs underline two forms of LTP in CA1 hippocampus in vivo. J Neurophysiol 1999;82:736-40.
- Kavalali ET, Hwang KS, Plummer MR. cAMP-dependent enhancement of dihydropyridine-sensitive calcium channel availability in hippocampal neurons. J Neurosci 1997;17:5334-48.
- Macdonald TL, Martin RB. Aluminum ion in biological systems. Trends Biochem Sci 1988;13:15-19.
- Le Bars PL, Katz MM, Berman N, Itil TM, Freedman AM, Schatzberg AF. A placebo-controlled, doubled-blind, randomized trial of an extract of Ginkgo biloba for dementia. J Am Med Assoc 1997;278:1327-32.
- Lenoir M, Pedruzzi E, Rais S, Drieu K, Perianin A. Sensitization of human neutrophil defense activities through activation of platelet-activating factor receptors by ginkgolide B, a bioactive component of the Ginkgo biloba extract EGB 761. Biochem Pharmacol 2002;63:1241-9.
- Bastianetto S, Zheng WH, Quirion R. The Ginkgo biloba extract (EGb 761) protects and rescues hippocampal cells against nitric oxide-induced toxicity: involvement of its flavonoid constituents and protein kinase C. J Neurochem 2000;74:2268-77.
- Zhu L, Wu J, Liao H, Gao J, Zhao XN, Zhang ZX. Antagonistic effects of extract from leaves of Ginkgo biloba on glutamate neurotoxicity. Acta Pharmacol Sin 1997;18:344-7.
- Kondratskaya EL, Lishko PV, Chatterjee SS, Krishtal OA. BN52021, a platelet activating factor antagonist, is a selective blocker of glycine-gated chloride channel. Neurochem Int 2002;40:647-53.
- Chatterjee SS, Kondratskaya EL, Krishtal OA. Structure- activity studies with Ginkgo biloba extract constituents as receptor-gated chloride channel blockers and modulators. Pharma-copsychiatry 2003;36:S68-77.