Interferon-α enhances sensitivity of human osteosarcoma U2OS cells to doxorubicin by p53-dependent apoptosis1
Introduction
Osteosarcoma is the most common primary malignant tumor of bone[1]. The current treatment is the combination of surgery and neoadjuvant chemotherapy using multidrugs, including methotrexate, doxorubicin, cisplatin and cyclophosphamide[2]. Although chemotherapy significantly increases patient survival, there is no effective treatment for metastatic osteosarcoma. The frequent acquisition of drug-resistant phenotypes and unwanted side-effects are often associated with chemotherapy and remain a serious problem[3]. Therefore, novel therapeutic strategies to increase chemosensitivity need to be developed.
Interferonα (IFN)-α, which belongs to the type I IFN, is a multifunctional cytokine exerting immunomodulatory, antiviral, and anticancer effects[4-6]. It interacts with the IFN-α/β receptor on the cell surface to induce the activation of JAK/STAT1 (Janus activated kinase/signal transducers and activators of transcription 1) pathway to regulate the transcription of the genes controlling antiproliferative activities[6].
p53 is a famous tumor suppressor gene which is commonly destroyed by mutation or deletion in malignant tumors including osteosarcoma[7]. It controls cell cycle arrest and apoptosis induced by chemotherapeutic agents including doxorubicin, by activating Bax, p21, PUMA (p53 Upregulated Modulator of Apoptosis) and Noxa, which are target genes of p53[8]. Recently, p53 was found to be correlated with Type I IFN-induced apoptosis in human cancer cells[9,10]. Thus we hypothesize that IFNá may cooperate with chemotherapeutic drugs to enhance antitumor effects by modulating p53-dependent apoptosis. To test this hypothesis, we utilized the p53-wild and p53-mutant osteosarcoma cells to examine whether IFNα increases doxorubicin sensitivity to determine its molecular mechanism. We report that IFNα enhances doxorubicin sensitivity in osteosarcoma p53-wild U2OS, but not p53-mutant MG63 cells and define for the first time a p53-dependent apoptosis as the molecular mechanism. This work also supports the view that the proper combination of IFNα and conventional chemotherapeutic agents may be a rational strategy for improving the treatment of osteosarcoma with functional p53.
Materials and methods
Cell culture The human osteosarcoma U2OS cells[11] containing wild p53 and MG63 cells[12] containing mutant p53 were maintained in DMEM (Gibco BRL, Grand Island, NY, USA) and supplemented with 10% fetal bovine serum, 100 U/mL penicillin, and 100 µg/mL streptomycin at 37 oC in a 5% CO2 humidified atmosphere.
Drugs and reagents Doxorubicin, Hoechst 33258 and MTT were obtained from Sigma (St Louis, MO, USA). Human recombinant IFNα2a were purchased from Peprotech (Rocky Hill, NJ, USA).
MTT assays The logarithmically growing U2OS and MG63 cells were seeded in 96-well plate at a density of 5×103 cells/well. After overnight growth, the cells were treated with IFNα and doxorubicin, alone or in combination. After the indicated time courses, 10 µL of 5 mg/mL MTT was added into each well followed by incubation for an additional 4 h. The supernatants were removed and 200 µL DMSO was added. After the crystals had dissolved, absorbance at 450 nm was measured in the microplate reader.
Flow cytometry analysis The cells were collected, washed twice with ice-cold phosphate-buffered saline (PBS), resuspended in cold PBS, and fixed with 70% ethanol. After fixation overnight and subsequent rehydration in PBS for 30 min at 4 oC, the samples were stained for 30 min in the dark with 50 µg/mL propidium iodide (Sigma, USA) containing 125 units/mL protease-free RNase, both diluted in PBS in a flow cytometer (Beckman Coulter, Fullerton, CA, USA).
Morphological analysis of apoptosis The cells were collected by centrifugation at 1000×g for 5 min, washed twice with PBS, and stained with 10 µg/mL Hoechst 33258 (Sigma, USA) for 15 min, followed by examination using a Olympus fluorescence microscope (Olympus, Shinjuku-ku, Tokyo, Japan).
DNA fragmentation assay The cells (3×106) were collected and washed once with PBS. DNA was extracted using DNAZol reagent (Invitrogen, Carlsbad, CA, USA) according to the manufacturer’s instructions and electrophoresed on 2% agarose gel containing 0.5 µg/mL ethidium bromide. The gel was photographed with UV illumination.
Western blot analysis The cells were collected and lysed with the lysis buffer [10 mmol/L Tris-HCl (pH 7.4), 5 mmol/L MgCl2, 1 mmol/L EDTA, 25 mmol/L NaF, fresh 100 µmol/L Na3VO4 and l mmol/L dithiothreitol]. An equal amount of protein determined by Bradford assay was resolved by SDS-PAGE and transferred onto polyvinylidene difluoride (PVDF) membranes (Roche, Grenzacherstrasse, Basel, Switzerland). The blots were incubated with primary antibody overnight at 4 oC, followed with 3 washes in TBST [20 mmol/L Tris-HCl (pH 7.6), 150 mmol/L NaCl, 0.1% Tween-20] for 5 min, and then incubated with horseradish peroxidase-conjugated secondary antibody for 1 h at room temperature. Signals were detected using enhanced chemiluminescence detection system (Amersham Pharmacia Biotech, Piscata-way, NJ, USA) and exposed to X-ray film (Kodak, Shanghai, China). All the antibodies were obtained from Santa Cruz Biotechnology (Santa Cruz, CA, USA).
RT-PCR Total RNA was extracted with TRIZol reagent (Invitrogen, Carlsbad, CA, USA) according to the manufac-turer’s recommendations. For the RT reaction, 2 µg RNA was combined with 0.5 µg oligo (dT)15 (15 µL total volume). The mixture was incubated at 70 oC for 5 min and placed on ice. Then 5 µL 5× M-MLV (moloney murine leukemia virus) reaction buffer, 1.25 µL 4× dNTP (10 mmol/L), 1 µL M-MLV (Promega, Madison, WI, USA; 200 U/µL), 0.625 µL RNaseOUT (40 U/µL) was added (25 µL total volume). The tube was incubated at 42 oC for 60 min and then at 75 oC for 10 min for termination. The PCR reaction was performed in the presence of Taq DNA polymerase, dNTP mix, and PCR buffer primers (all from Invitrogen, USA). After denaturation at 94 oC for 2 min, the samples underwent 30 cycles of amplification (1 min at 94 oC, 1 min at 58 oC for p53 or 55 oC for β-actin, and 1 min at 72 oC) with a 10 min extension at 72 oC following the last cycle. The sense and antisense primers were: 5'-CAG CCA AGT CTG TGA CTT GCA CGT AC-3' and 5'-CTA TGT CGA AAA GTG TTT CTG TCA TC-3' for p53 and 5'-ACT ACC TCA TGA AGA TCC TC-3' and 5'-CTA AAG ATT GCG TGG CGA GG-3' for β-actin. Products were electrophoresed on 1.5% agarose gels containing 0.5 µg/mL ethidium bromide.
Small interfering RNA transfection The U2OS cells were plated onto 6-well plates at a density of 3×105 cells/well with growth medium without antibiotics. After overnight incubation, transfection was performed at a confluency of 50% by using Opti-MEM media (Invitrogen, Carlsbad, CA, USA), Lipofectamine 2000 (Invitrogen, Carlsbad, CA, USA), and specific or non-specific siRNA for p53 according to the manufacturer’s recommendations. Six hours later, the medium was replaced with growth medium without antibiotics. After transfection for 20 h, the cells were trypsinized and sub-seeded onto 96 well plates. After incubation for another 4 h, the cells were treated as described for the MTT assay. The p53 siRNA duplexes were synthesized by Genepharma (Shanghai, China). The mRNA sequence to be targeted by p53-siRNA was 5'-CTA CTT CCT GAA AAC AAC G-3'.
Results
IFNα enhances doxorubicin-induced cytotoxicity in p53-wild U2OS, but not p53-mutant MG63 cells We first determined whether IFNα could enhance doxorubicin-induced cytotoxicity using MTT assay. After treatments with IFNα, doxorubicin or both for 72 h, the proliferation of the U2OS cells was not found to be inhibited by IFNα and was slightly suppressed by doxorubicin. However, doxorubicin-induced cytotoxicity was significantly enhanced by IFNα (Figure 1). In contrast, such effects were not observed in p53-mutant osteosarcoma MG63 cells (Figure 1). The MTT results show that IFNα enhances doxorubicin-induced cytotoxicity in p53-wild U2OS, but not p53-mutant MG63 cells. The results indicate that p53 may contribute to this phenomenon.
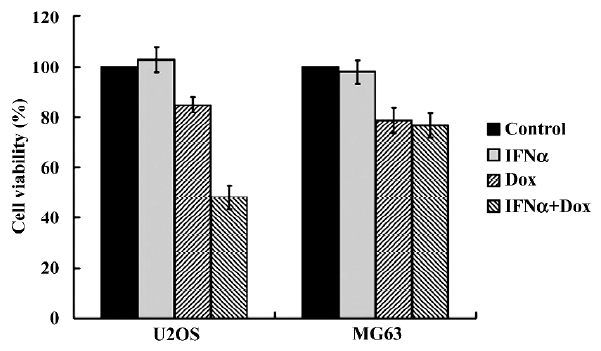
IFNα enhances doxorubicin-induced apoptosis in p53-wild U2OS, but not p53-mutant MG63 cells We next used flow cytometry analysis to evaluate the effect of IFNα on doxorubicin-induced apoptosis in U2OS and MG63 cells. The fraction of sub-G1 cells is a dependable way of determining cells undergoing apoptosis[13]. IFNα did not induce obvious apoptosis, but notably increased doxorubicin-induced apoptosis in p53-wild U2OS cells (Figure 2A), but not p53-mutant MG63 cells (Figure 2B). Moreover, Hoechst33258 staining, which stains nuclei to manifest apoptotic morphological change, revealed a higher level of nuclear condensation and fragmentation in the U2OS cells treated for 72 h with the IFNα/doxorubicin combination than either alone (Figure 3A). We next used agarose gel electrophoresis to further confirm apoptosis. The internucleosomal DNA fragmentation was observed in the U2OS cells treated with the IFNα/doxorubicin combination for 72 h, compared with either alone (Figure 3B). In contrast, such results were not obtained for the p53-mutant MG63 cells (data not shown). These results from different methods strongly indicated that IFNα enhanced doxorubicin-induced apoptosis in p53-wild U2OS, but not p53-mutant MG63 cells.
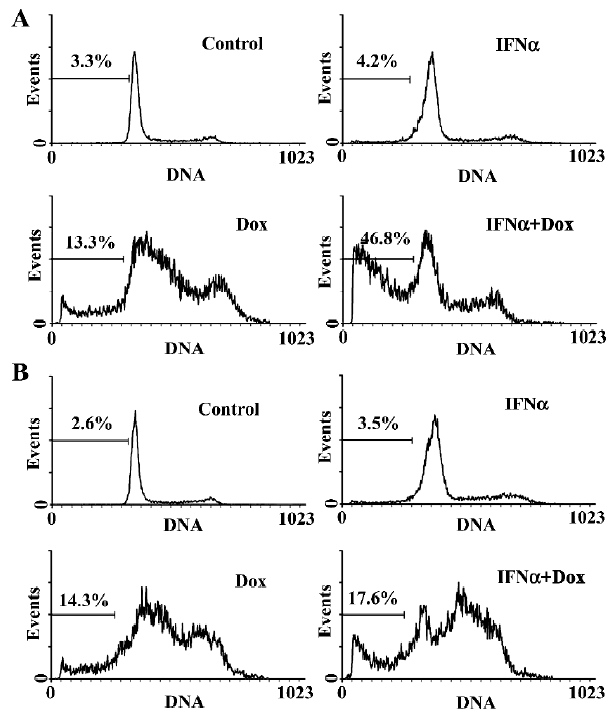
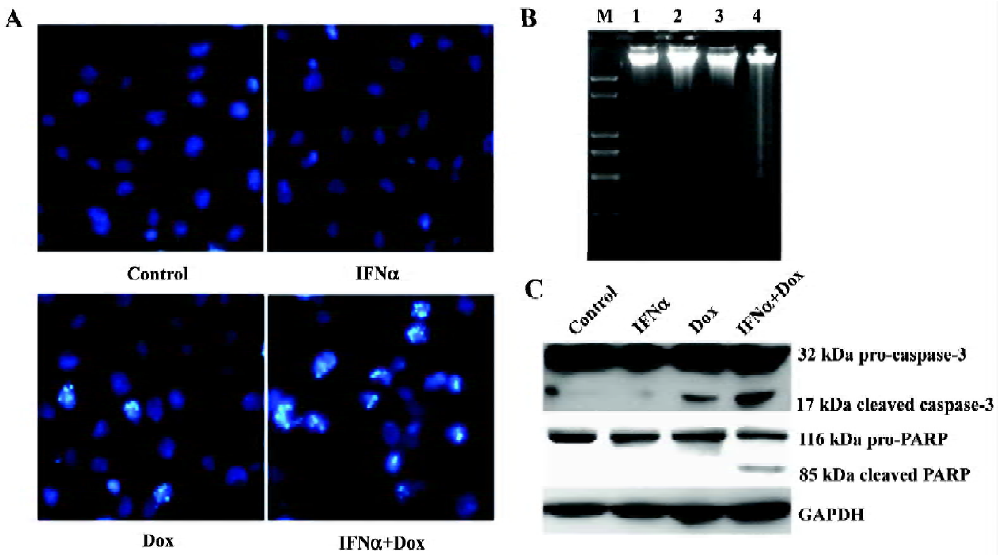
Caspase-3 is a key executor of apoptotic cell death signals by selectively cleaving proteins, such as poly (ADP-ribose) polymerase (PARP). Both caspase-3 activation and PARP cleavage are hallmarks of apoptosis. We next used Western blotting for their detection them. Caspase-3 and PARP were cleaved to yield 17 and 85 kDa fragments in response to the IFNα/doxorubicin combination in the U2OS cells, respectively (Figure 3C).
IFNα activates p53 as a transcription factor in response to doxorubicin in osteosarcoma U2OS cells To determine whether IFNα affects the doxorubicin-induced activation of the p53 pathway, Western blotting was applied to examine the expression of p53 and well-known transcriptional target genes of p53, such as Bax, Bcl-2, Mdm2, and p21. The p53 protein level was unaltered by IFNα, but was enhanced by doxorubicin and further augmented by the IFNα/doxorubicin combination. The expression of pro-apoptotic Bax and p21 was further increased by the IFNα/doxorubicin combination than either alone. The Mdm2 expression level was also increased. Adversely, the expression of anti-apoptotic Bcl-2 was decreased by the IFNα/doxorubicin combination compared with either alone (Figure 4A). In contrast, such results were not observed in the MG63 cells (Figure 4A). In addition, RT-PCR showed that the p53 mRNA level was increased greatly by doxorubicin, but was not further augmented by the IFNα/doxorubicin combination in the U2OS cells (Figure 4B), suggesting that the combination-induced p53 protein upregulation was mediated in a post-transcriptional manner.
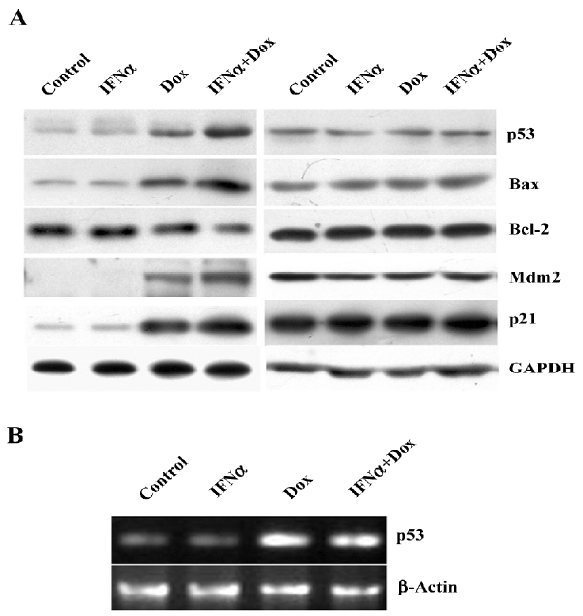
p53 silencing mediated by small interfering RNA in U2OS cells results in the decrease of cytotoxicity and apoptosis induced by the IFNα/doxorubicin combination To study the presence of the IFNα/β receptor in U2OS and MG63 cells, Western blotting was used to detect its expression. The IFNα/β receptor was found to be expressed equally in both cell lines (Figure 5A). To examine whether p53 was required for this enhanced apoptosis, we next used p53-specific siRNA transfection to suppress p53 expression in the U2OS cells. p53 siRNA effectively inhibited p53 protein expression in the U2OS cells treated with the IFNα/doxorubicin combination (Figure 5B). This p53 silencing significantly reduced the combination-induced cell death (Figure 5C). Furthermore, as key markers of apoptosis, caspase-3 activation and PARP cleavage were also completely suppressed in U2OS cells where p53 expression had been suppressed, compared with the nonspecific control (Figue 5D). These results show that the enhanced apoptosis induced by the IFNα/doxorubicin combination was p53-dependent.
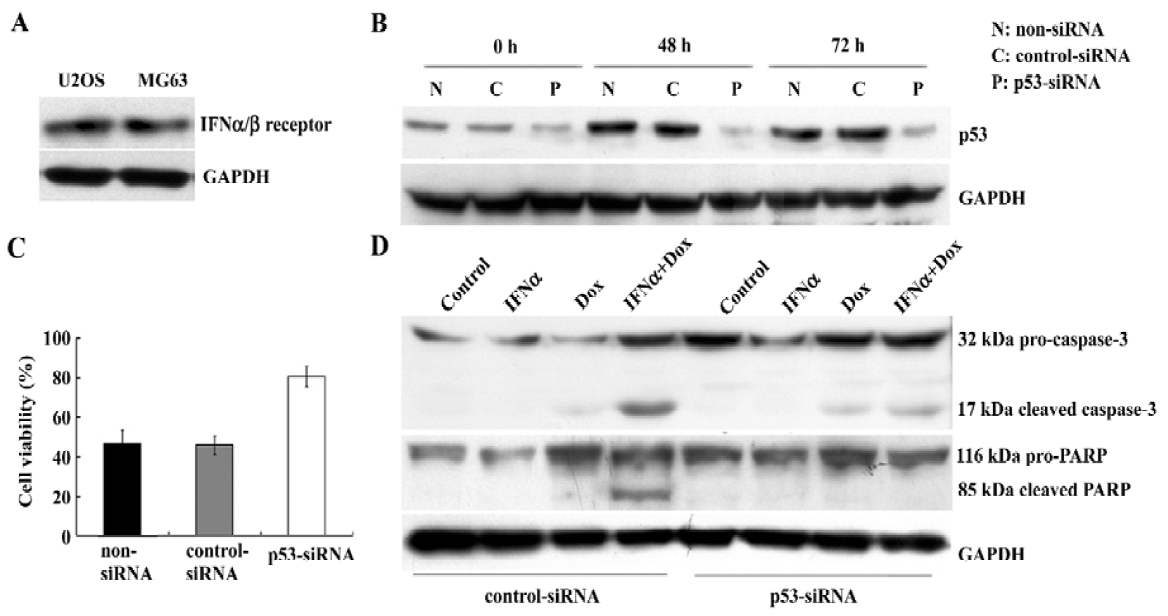
Discussion
IFNα is an approved treatment option for tumor therapy, however; biological activity remains elusive. For example, IFNα induces cell cycle arrest, triggers apoptosis, and increases chemotherapy-induced cytotoxicity in specific cancer cells[14-16]. Recently, IFNα was reported to directly suppress the growth of some osteosarcoma cells[17]. However, the role of IFNα in the chemosensitivity of human osteosarcoma is largely unknown. In the present work, we showed that IFNα alone unaltered cell growth but promoted doxorubicin-induced cytotoxicity pronouncedly in osteosarcoma U2OS cells containing wild p53. Furthermore, the enhanced cytotoxicity was demonstrated to be mediated by apoptosis using FACS, Hoechst 33258 staining, DNA fragmentation, caspase-3 activation and PARP cleavage. In contrast, such effects were not observed in the p53-mutant MG63 cells. Although IFNα can directly induce apoptosis in a large group of tumor cells, such an effect was not observed in the U2OS and MG63 cells. This is not unusual because these 2 cell lines are both null of ARF (Alternative Reading Frame) [18], which is required for IFNα-induced apoptosis in specific cell types[19].
Although neoadjuvant chemotherapy shows a promising efficacy in treating osteosarcoma, the resistance against chemotherapy and drug-induced side-effects remain serious problems[2]. The topoisomerase II inhibitor doxorubicin is an antitumor drug widely used in treating human osteosarcoma[1]. Topoisomerase II is a nuclear enzyme that functions during both DNA replication and transcription[2]. Doxorubicin is able to induce DNA damage and leads to cell cycle arrest or apoptosis by activating p53[2]. As a tumor suppressor gene which is often disrupted in human malignancies including osteosarcoma[8,20], the p53 gene product is also involved in type I IFN-induced apoptosis[9,10]. Based on these findings and our result that the enhanced apoptosis occurred in the p53-wild U2OS, but not p53-mutant cells, we hypothesize that p53 may contribute to the IFNα/doxorubicin combination-induced apoptosis. Our results showed that the enhanced apoptosis in response to IFNα/doxorubicin combination in the U2OS cells was associated with an accumulation of the p53 protein, which confirmed our hypothesis.
The Mdm2-p53 feedback loop is the main mechanism in the regulation of the p53 level[21]. Mdm2, another transcriptional target of p53, inhibits p53 by directly binding to it to antagonize its activity and enhance its degradation. Adversely, when the nuclear p53 level is elevated, it activates the transcription of the Mdm2 gene[21]. After treatment with the combination, Mdm2 expression was consistent with p53 upregulation. This is possibly because p53 upregulation induced Mdm2 expression according to this negative feedback loop. However, the p53 mRNA level did not further increase following the IFNα/doxorubicin combina-tion, suggesting that p53 upregulation is mediated in a post-transcriptional manner. However, the exact mechanism is not clear.
Bax and Bcl-2, members of the Bcl-2 family, exert pro-apoptotic or anti-apoptotic functions respectively to regulate p53-dependent apoptosis[22]. The Bcl-2 protein is able to repress a number of apoptotic death programs[23]. The 21 kDa protein partner Bax, which overexpresses to counter the death repressor activity of Bcl-2, can enhance apoptosis. The ratio of Bcl-2 to Bax determines survival or death following an apoptotic stimulus[23-24] In this study, the combination subsequently increased Bax and decreased Bcl-2 expres-sion resulting from p53 upregulation. The wild-type p53 gene is a negative regulator of cell growth by the transcriptional activation of p21 which plays a crucial role in controlling DNA repair, cell differentiation, and apoptosis in response to p53 activation[25]. Furthermore, the expression of p21 was also further increased by the combination. These events may induce the mitochondrial permeability transition, which can release cytochrome c and culminate in apoptotic cell death[22].
Caspase-3 is a key executor of the apoptotic machinery[26]. Once activated by apoptotic signals, caspase-3 is proteolytically cleaved to active its substrates, such as PARP, resulting in the activation of the DNA fragmentation of apoptosis[27]. Our study showed that IFNα markedly promoted caspase-3 activation and PARP cleavage in doxorubicin-treated U2OS cells, suggesting that a caspase-3 activation pathway was involved.
Although we proved that p53 activation is involved in enhanced apoptosis induced by the IFNα/doxorubicin combination, whether it is required for this effect remains unclear. We show here that siRNA-mediated p53 knockdown markedly decreased the apoptotic response to the IFNα/doxorubicin combination, as determined by the decrease of caspase-3 activation and PARP cleavage, indicating that p53 function is required for the IFNα/doxorubicin combination-induced cytotoxicity and apoptosis.
In conclusion, we demonstrated that IFNα enhanced the sensitivity of human osteosarcoma U2OS cells to doxorubicin by apoptosis, and defined a p53-dependent pathway as the underlying mechanism. The combination of IFNα and standard chemotherapeutic agents may help to achieve enhanced chemosensitivity and reduce the side-effects in treating osteosarcoma with functional p53.
References
- Daw NC, Billups CA, Rodriguez-Galindo C, McCarville MB, Rao BN, Cain AM, et al. Metastatic osteosarcoma. Cancer 2006;106:403-12.
- Bruland OS, Pihl A. On the current management of osteosarcoma. A critical evaluation and a proposal for a modified treatment strategy. Eur J Cancer 1997;33:1725-31.
- de Saint Aubain Somerhausen N, Fletcher CD. Soft-tissue sarcomas: an update. Eur J Surg Oncol 1999;25:215-20.
- Prejean C, Colamonici OR. Role of the cytoplasmic domains of the type I interferon receptor subunits in signaling. Semin Cancer Biol 2000;10:83-92.
- Mogensen KE, Lewerenz M, Reboul J, Lutfalla G, Uze G. The type I interferon receptor: structure, function, and evolution of a family business. J Interferon Cytokine Res 1999;19:1069-98.
- Stark GR, Kerr IM, Williams BR, Silverman RH, Schreiber RD. How cells respond to interferons. Annu Rev Biochem 1998;67:227-64.
- Chandar N, Billig B, McMaster J, Novak J. Inactivation of p53 gene in human and murine osteosarcoma cells. Br J Cancer 1992;65:208-14.
- Levine AJ, Momand J, Finlay CA. The p53 tumour suppressor gene. Nature 1991;351:453-6.
- Porta C, Hadj-Slimane R, Nejmeddine M, Pampin M, Tovey MG, Espert L, et al. Interferons alpha and gamma induce p53-dependent and p53-independent apoptosis, respectively. Oncogene 2005;24:605-15.
- Takaoka A, Hayakawa S, Yanai H, Stoiber D, Negishi H, Kikuchi H, et al. Integration of interferon-alpha/beta signalling to p53 responses in tumour suppression and antiviral defence. Nature 2003;424:516-23.
- Ponten J, Saksela E. Two established in vitro cell lines from human mesenchymal tumours. Int J Cancer 1967;2:434-47.
- Heremans H, Billiau A, Cassiman JJ, Mulier JC, de Somer P. In vitro cultivation of human tumor tissues. II. Morphological and virological characterization of three cell lines. Oncology 1978;35:246-52.
- Ormerod MG. The study of apoptotic cells by flow cytometry. Leukemia 1998;12:1013-25.
- Roos G, Leanderson T, Lundgren E. Interferon-induced cell cycle changes in human hematopoietic cell lines and fresh leukemic cells. Cancer Res 1984;44:2358-62.
- Rodriguez-Villanueva J, McDonnell TJ. Induction of apoptotic cell death in non-melanoma skin cancer by interferon-alpha. Int J Cancer 1995;61:110-4.
- Kondo M, Nagano H, Wada H, Damdinsuren B, Yamamoto H, Hiraoka N, et al. Combination of IFN-alpha and 5-fluorouracil induces apoptosis through IFN-alpha/beta receptor in human hepatocellular carcinoma cells. Clin Cancer Res 2005;11:1277-86.
- Manara MC, Serra M, Benini S, Picci P, Scotlandi K. Effectiveness of Type I interferons in the treatment of multidrug resistant osteosarcoma cells. Int J Oncol 2004;24:365-72.
- Park YB, Park MJ, Kimura K, Shimizu K, Lee SH, Yokota J. Alterations in the INK4a/ARF locus and their effects on the growth of human osteosarcoma cell lines. Cancer Genet Cytogenet 2002;133:105-11.
- Sandoval R, Xue J, Pilkinton M, Salvi D, Kiyokawa H, Colamonici OR. Different requirements for the cytostatic and apoptotic effects of type I interferons. Induction of apoptosis requires ARF but not p53 in osteosarcoma cell lines. J Biol Chem 2004;279:32275-80.
- Toguchida J, Yamaguchi T, Ritchie B, Beauchamp RL, Dayton SH, Herrera GE, et al. Mutation spectrum of the p53 gene in bone and soft tissue sarcomas. Cancer Res 1992;52:6194-9.
- Moll UM, Petrenko O. The MDM2-p53 interaction. Mol Cancer Res 2003;1:1001-8.
- Cory S, Adams JM. The Bcl2 family: regulators of the cellular life-or-death switch. Nat Rev Cancer 2002;2:647-56.
- Hockenbery DM, Oltvai ZN, Yin XM, Milliman CL, Korsmeyer SJ. Bcl-2 functions in an antioxidant pathway to prevent apoptosis. Cell 1993;75:241-51.
- Thornborrow EC, Patel S, Mastropietro AE, Schwartzfarb EM, Manfredi JJ. A conserved intronic response element mediates direct p53-dependent transcriptional activation of both the human and murine bax genes. Oncogene 2002;21:990-9.
- el-Deiry WS, Harper JW, O’Connor PM, Velculescu VE, Canman CE, Jackman J, et al. WAF1/CIP1 is induced in p53-mediated G1 arrest and apoptosis. Cancer Res 1994;54:1169-74.
- Haupt S, Berger M, Goldberg Z, Haupt Y. Apoptosis - the p53 network. J Cell Sci 2003;116:4077-85.
- Harris SL, Levine AJ. The p53 pathway: positive and negative feedback loops. Oncogene 2005;24:2899-908.