Recombinant adeno-associated virus-mediated delivery of antisense angiotensin II receptor 1 gene attenuates hypertension development1
Introduction
Epidemiological data have suggested a correlation between dietary salt and blood pressure regulation in the prevalence and progression of essential hypertension, although the salt-blood pressure theory has remained the subject of ongoing controversy in a number of studies[1–4]. It has been demonstrated that the renin-angiotensin (RAS) is important in the development and maintenance of hypertension in both essential hypertensive patients and animal models of hypertension[5,6]. Moreover, many studies have revealed that high salt could stimulate the RAS and induce angiotensin II receptor 1 (AT1R) overexpression, which may be responsible for the elevation of blood pressure[7,8]. The blockade or interruption of RAS by pharmacological agents, such as the angiotensin-converting enzyme inhibitor and AT1R antagonists, have been extremely successful in the treatment of hypertension and the prevention of it complications. The findings indicate that the RAS components are crucial for the development of hypertension and cardiovascular function.
Gene therapy is a promising strategy for the treatment of hypertension[9]. Applications of antisense RAS genes to block the expression of either angiotensinogen or AT1R have demonstrated efficiency of this therapeutic strategy. Iyer et al reported that retroviral vector-mediated antisense AT1R cDNA gene delivery resulted in a chronic reduction up to 30–60 mmHg in hypertension in spontaneously hypertensive rats (SHR) rather than Wistar Kyoto normotensive rats[10]. The intracerebroventricular application of antisense oligonucleotides, directed to either AT1R or angiotensinogen mRNA also significantly reduced blood pressure in hypertensive animals with a single injection[11–13]. Moreover, an injection of antisense cDNA or oligonucleotides of the angiotensin-converting enzyme, renin, or angiotensinogen genes also induced a reduction of blood pressure in hypertensive animals[14–17]. All these findings suggest that blocking RAS genes may serve as target or candidate genes for hypertension gene therapy.
Another concern is which vector could be used to carry genes for chronic or permanent reduction in blood pressure. Several viral vectors, including retroviruses, lentivirus virus, and recombinant adeno-associated virus vector (rAAV) can be adaptive for gene therapy of genetic or chronic diseases. However, the retrovirus vector should not be considered due to the carcinogenic effects. rAAV, which either integrates into the genome or remains in the nucleus as a stable episome, offers the most attractive advantages and may be the most promising gene vector for long-term therapy[18,19]. The adeno-associated virus (AAV) is safe to use. It does not induce any pathogenic responses and does not replicate inside cells. The AAV is a defective parvovirus which cannot replicate in cells without the presence of a wild-type adenovirus[20,21]. The AAV is effective as a vector as it does not produce viral proteins that stimulate inflammatory reactions and contains sufficient carrying capacity for the insertion of an antisense cDNA for AT1R. More recently, a new double-stranded rAAV was developed, and this double strand can express more rapidly and efficiently than a single-strand rAAV, which makes it more attractive[22]. In the present study, the rAAV was used to carry the rat antisense AT1R gene (rAAV-AT1-AS) for hypertension therapy. It was demonstrated that the rat rAAV-AT1-AS delivery via intravenous injection prevented the long-term, salt-induced development of blood pressure and attenuated cardiovascular complications of hypertension.
Materials and methods
Preparation of rAAV-AT1 -AS and rAAV-GFP (green fluorescent protein, as control) The rAAV-D(+) vector (double-stranded rAAV Vector plasma), the adenovirus helper plasmid pXX6, and the packaging plasmid pXX2 have been described previously[21]. A 660 bp fragment of rat vascular AT1R cDNA (127–786) was amplified with RT-PCR from rat kidney total RNA and ligated to the rAAV-D(+) vector in the antisense orientation after being identified by sequencing. The rAAV-AT1-AS and rAAV-GFP vector plasmids were constructed by inserting the rat AT1 antisense cDNA and GFP cDNA into the rAAV-D(+) vector driven by the cytomegalovirus promoter at BamHI and NotI sites, respectively. The vectors for rAAV-AT1-AS and rAAV-GFP were prepared using a triple plasmid cotransfection method in 293 cell lines, as described previously[21,23]. A total of 50 µg plasmid DNA per 15 cm plate (rAAV-AT1-AS or rAAV-GFP vector plasmids /pXX2/pXX6 were 1:1:1 molar ratios) were used for transfection. For large-scale rAAV preparations, 40×15 cm plates, each containing 5×106 293 cells were used, and a single-step gravity-flow column purification method was carried out, as previously described[24]. The eluted rAAV was aliquoted and stored at -80 °C. To determine the titer of the AAV vectors, 5 µL of the rAAV vector was digested with 5 U DNase I at 37 °C for 1 h followed by digestion with 100 µg proteinase K in proteinase K buffer for 1 h at 37 °C. The reaction was then extracted with an equal volume of phenol/chloroform/isoamyl alcohol, and the titer of rAAV-AT1-AS and rAAV-GFP was determined by dot blot hybridization with a 32P-labeled probe[23].
Animals Male Sprague-Dawley (SD) rats weighing 250–270 g were obtained from the Tongji Medical College Animal Center (Wuhan,Hubei Province, China). All of the animals were housed at room temperature with 12 h light/dark cycles and allowed free access to normal rat chow and water ad libitum. The experimental protocols complied with the National Institutes of Health Guidelines for the Care and Use of Laboratory Animals and were approved by the Chinese Academy of Sciences. The animals were randomly allocated to the groups of rAAV-AT1-AS, rAAV-GFP and Saline.
Gene delivery The animals were anesthetized intraperitoneally with pentobarbital at a dose of 40 mg/kg body weight. rAAV-AT1-AS, rAAV-GFP (about 2×1010 virion particles), or an equal volume of saline was then administered into the SD rats by tail vein injection. After the injection, the rats were kept warm under an infrared lamp until they recovered. After 2 weeks, the animals of rAAV-AT1-AS and rAAV-GFP groups were exposed to a high-salt diet (8% NaCl) and the animals in saline group received normal diet for 12 weeks, and then cardiac catheterization was performed for the hemodynamic measurements before the animals were killed under pentobarbital anesthesia (40 mg/kg body weight). The hearts, lungs, brains, aorta, and livers were harvested, snap-frozen in liquid nitrogen, and stored at -80 °C.
Blood pressure measurements The systolic blood pressure of the rats was measured with a manometer–tachometer (Rat tail NIBP system, ADI Instruments, Bella Vista, NSW, Australia) by cuffing the tails of the animals. The rats were restrained in a plastic cage for approximately 15–30 min to acclimatize and were minimally warmed before the blood pressure determination. Five to ten stable readings of systolic blood pressure were taken weekly for each animal. All of the measurements were performed between 21:00 and 24:00. The systolic blood pressure was represented by the mean of these stable recordings.
Serum and urine collection Urine samples were collected for 24 h in metabolic cages with 500 µL toluene to prevent the decay of the urine. The samples were stored at -80 °C before the urinary microalbumin concentration was measured.
Whole heart and kidney weight measurements At 12 weeks after the high-salt diet, the animals were anesthetized with pentobarbital and the hearts were removed. The whole heart, double kidney, and body were then weighed. The ratios of whole heart/body weight and double kidney/body weight were calculated.
RT-PCR analysis of rat AT1 mRNA Total RNA was extracted from fresh rat tissues using TRIZol reagent (Gibco, Carlsbad, CA, USA), according to the manufacturer’s instructions. RT-PCR for the rat AT1 transcription level was then performed using the RT-PCR kit (TaKaRa Biotechnology, Dalian, Liaoning, China), according to the manufacturer’s instructions. PCR primers specific for rat AT1 were 5'-GGA TGG CGG GAA GTC TAT T-3' for upstream and 5'-TGG GAA TCT TTG AGA ACT GG-3'for downstream (1151–1651 bp, which will not amplify transected antisense cDNA and RNA) and β-actin 5'-TCC TCC CTG GAG AAG AGC TA-3' for upstream and 5'-TCA GGA GGA GCA ATG ATC TTG-3' for downstream.
Urinary microalbumin and sodium measurement Urinary microalbumin was measured by ELISA. The albumin antibody-coated and blocked microtiter plates (96 wells), labeled with albumin antibody, albumin standard, and O-phenylenediamine(OPD) substrates were stored at 4 °C. The albumin standard (0.1–6.4 µg/mL) and rat urine samples were added to individual wells in a total volume of 50 µL dilution buffer. The labeled albumin antibody (50 µL) was added to the dilution buffer and mixed gently. The plates were incubated at 37 °C for 90 min, and after incubation, the plates were washed 4 times with wash solution. The color reaction was performed by adding 100 µL freshly prepared OPD substrate solution at 37 °C for 20 min. Then the plates were read at 490 nm on an Elx 800 ELISA reader (Biotek Instruments,Winooski, Vermont, USA). Urinary sodium was measured at the clinical laboratory of Tongji Hospital using an automatic analyzer.
Hemodynamic variables The hemodynamic variables were monitored using a catheter tip manometer (AD Instru-ments, Bella Vista, NSW, Australia) advanced from the right carotid artery via the aortic arch into the left ventricle (LV) at the end of the experiment. Global systolic function was measured as LV peak pressure (LVPSP), LV end-diastolic pressure (LVEDP), and the maximum–minimum rate of pressure increase (dp/dtmax). Global LV end-systole was defined as the point of minimum dp/dt and LV end-diastole as the beginning of the sharp upslope of the LV dp/dt tracing.
Analysis of morphology and collagen deposit The rat kidneys and hearts were preserved in 4% phosphate-buffered saline-formaldehyde solution and then embedded in paraffin. Four micrometer-thick sections were cut and stained with Sirius Red (collagen stained with Sirius Red), using the method described by Dobrzynski et al[25]. All of the sections were evaluated by investigators who were blind to the experi-ment. The percentage of extracellular matrix (ECM) production was quantified at the Department of Ultrastructural Pathology, Tongji Hospital with the use of the HAIPS pathological imagic analysis system (Wuhan, China). To quantify the ECM, red staining was expressed as a percentage of the total tissue area[26].
Statistical analysis The statistical significance of the difference in the systolic blood pressure between rats receiving rAAV-GFP and rats receiving rAAV-AT1-AS was determined by ANOVA. In addition, we used unpaired Student’s t-test to assess the differences in urine microal-bumin content, the ratios of total heart/body weight, and the results of the ECM quantification between the rAAV-GFP and rAAV-AT1-AS groups after gene delivery. The results were expressed as mean±SEM.
Results
Hypotensive effects of rAAV-AT1-AS The systolic blood pressure of all animals was monitored weekly before and after exposed to a high-salt diet. The results showed that a high-salt diet induced a significant elevation in the systolic blood pressure up to 132.3±12.9 mmHg (P<0.01. n=8. ANOVA) in the rAAV-GFP-treated rats compared with the rAAV-AT1-AS and saline solution-treated rats at 1 week. The systolic blood pressure kept elevating and the maximal blood pressure difference was up to 27.8 mmHg. In contrast, in the rAAV-AT1-AS-treated animals and normal controls, the blood pressure was stable for the duration of the experiment (12 weeks; Figure 1). These results indicated that rAAV-AT1-AS delivery prevents the development of high blood pressure in high-salt diet rats long term.
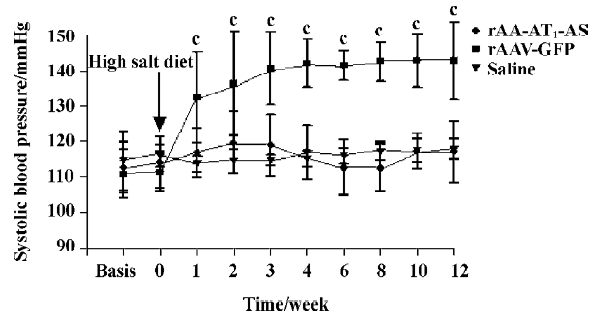
Hemodynamic variables The hemodynamic variables were monitored using a catheter tip manometer advanced from the right carotid artery via the aortic arch into the LV. First, the intra-arterial blood pressure was recorded (126.5±2.95 mmHg in the normal control, 129.2±7.72 mmHg in rAAV-AT1-AS vs 148.0±7.57 mmHg in rAAV-GFP, P<0.01, n=5) indicating consistency with tail artery blood pressure monitoring. The LVPSP, the left LVEDP, and the maximal/minimum ratio of LVP (±dp/dtmax) were measured and evaluated. The results showed that significant differences in hemodynamics between different groups were observed (Figure 2). In the rAAV-GFP-treated animals, the LVPSP (190.3±6.02 vs 167.5±4.94 mmHg, P<0.05, n=5), +dp/dtmax (9703.8±131.4 vs 7837±930.3 mmHg/s, P<0.05, n=5), and –dp/dtmax (5830.4±165.4 vs 4366±353.2 mmHg/s, P<0.05, n=5) were significantly lowered, and LVEDP increased (4.04±0.07 vs 5.01±0.13 mmHg, P<0.05, n=5) compared with the normal control. In contrast, rAAV-AT1-AS treatment prevented the deterioration of the cardiac function index (190.3±6.02, 9703.8±131.4, 5830.4±165.4, and 4.04±0.07, respectively) without significant differences compared with the normal control, but was significantly different compared with the rAAV-GFP-treated animals (P<0.05; Figure 2). These data indicate that rAAV-AT1-AS treatment significantly attenuates systolic and diastolic dysfunction in salt-induced, hypertensive rats as high blood pressure was reduced.
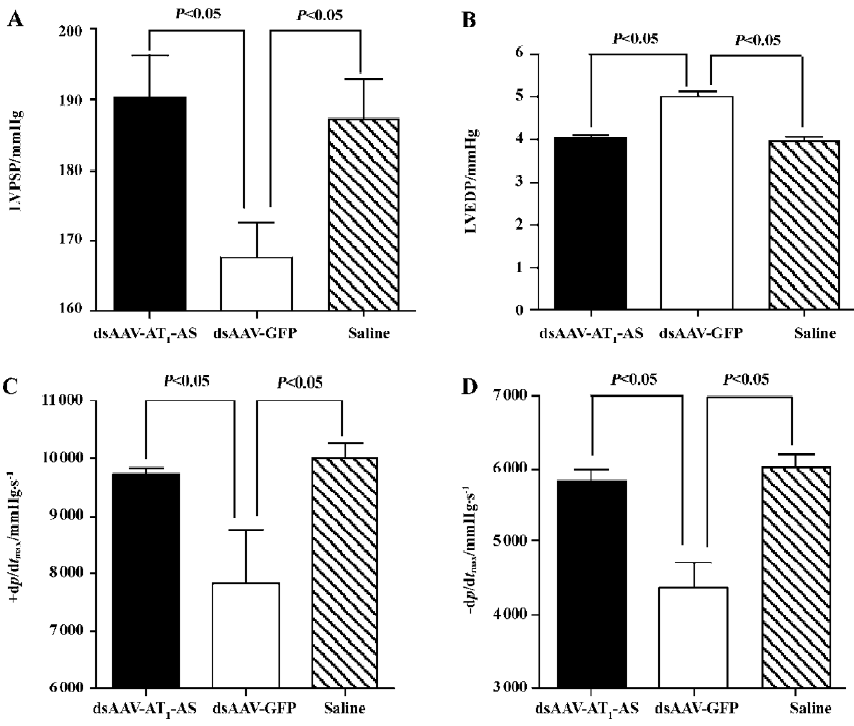
Attenuation of cardiovascular hypertrophy and fibrosis Myocardial hypertrophy is an important part of heart injury resulting from hypertension, and serves as an independent predictor of cardiovascular events in clinical practice. In this study, we measured the ratio of heart weight/body weight to analyze the effects of rAAV-AT1-AS treatment on myocardial hypertrophy and remodeling. As shown in Figure 3, this ratio was significantly reduced in the rAAV-AT1-AS-treated rats compared with the rAAV-GFP-treated animals (2678.1±105.5 vs 2904.2±34.4 mg/kg, n=8, P<0.05). Sirius Red staining for collagen I-VI, representing myocardial fibrosis and matrix proliferation, showed that the red-stained area significantly increased in the rAAV-GFP-treated animals compared with the rAAV-AT1-AS-treated rats and normal control groups, suggesting an increased collagen deposit in salt-induced, hypertensive rats (Figure 4). Meanwhile, the structures were deranged and the sizes of the cardiac myocytes were not uniform in the control rats in LV sections. A further quantitative analysis of the Sirius Red-stained sections was performed using HAIPS pathological imagic analysis system and was expressed as the ratio of collagen to the kidney area (percent-age of ECM). The results demonstrated that AT1-AS significantly reduced ECM accumulation compared with the rAAVGFP-treated animals (4.26±3.51 vs 15.58±6.25% ECM, n=8, P<0.05; Figure 4D).
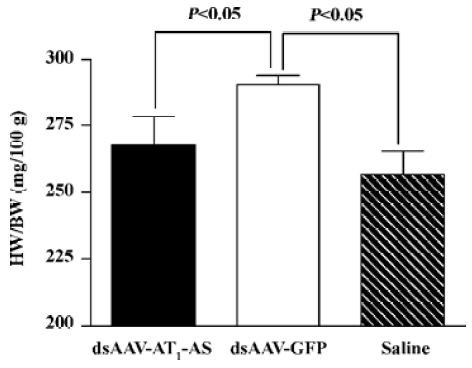
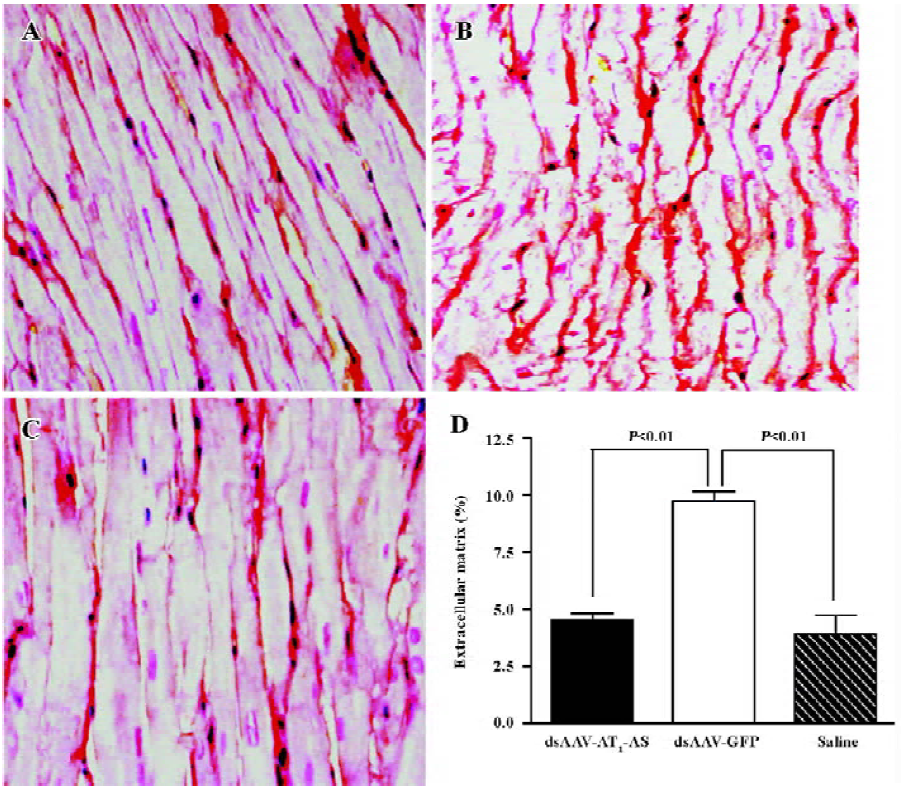
AT1-AS treatment decreases urinary microalbumin secretion An increased albumin concentration in the urine is a marker of kidney injury, as well as an independent predictor of cardiocerebral vascular disease. In this study, the micro-albumin level in the urine was measured by ELISA. Initially, the urine microalbumin levels had no difference between the 3 groups. However, after exposure to a high-salt diet, the urine microalbumin level in the control animals receiving rAAV-GFP reached 2.95 ±0.82 ng/mL and 3.05±0.76 ng/mL, respectively, at weeks 6 and 12), which was significantly higher than that in rats receiving rAAV-AT1-AS (2.65 ±0.69 ng/mL and 2.72±0.86 ng/mL at weeks 6 and 12, respectively, P<0.05) and saline injection (2.56±0.41 ng/mL and 2.52±0.58 ng/mL, at week 6 and 12, respectively, P<0.05, Figure 5). The results suggest that rAAV-AT1-AS treatment markedly attenuates the renal damage induced by hypertension from a high-salt diet.
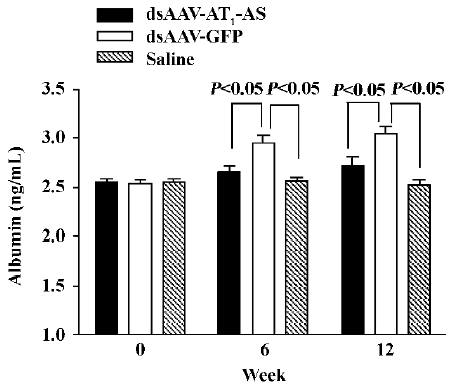
Protective effects of AT1-AS on kidneys To evaluate the beneficial effects of AT1-AS gene delivery in high-salt diet rats, collagen deposit in the kidneys was examined by Sirius Red staining. In the rAAV-GFP rats, there was more intense and diverse red staining in glomerules than that found in the AT1-AS-treated and normal control animals (Figure 6A–6C). The ECM accumulation was then quantified and was markedly lower in the group receiving rAAV-AT1-AS (11.80%±1.22%) than that in the rats receiving GFP vectors (16.22%±0.85%, P<0.05; Figure 6D). The results further indicate that AT1-AS treatment significantly attenuates renal damage induced by hypertension.
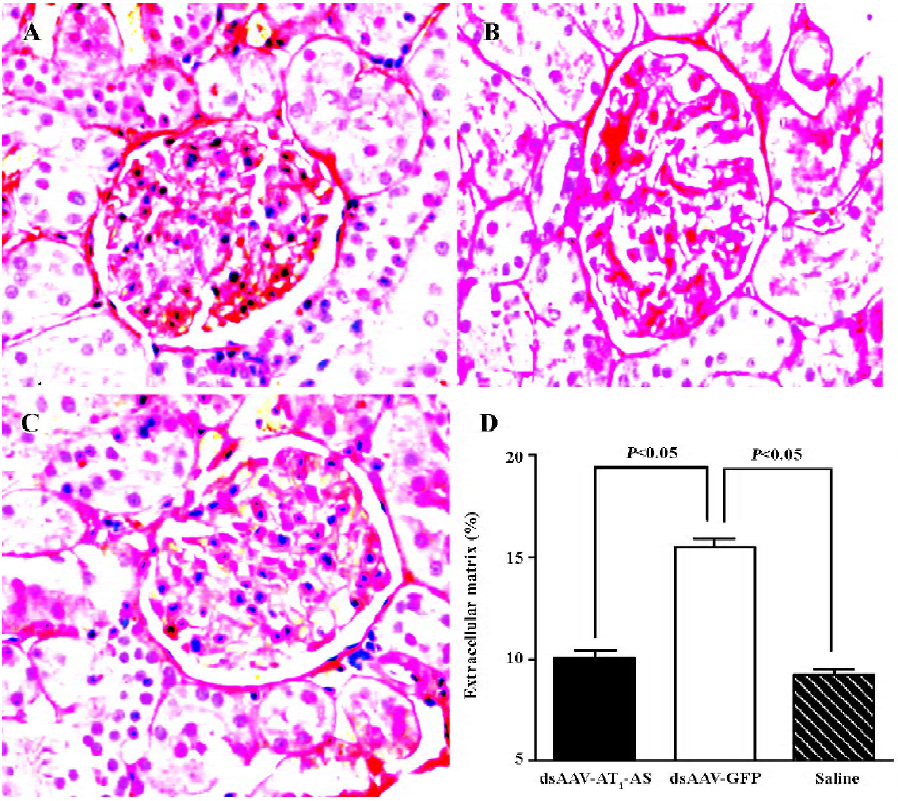
Expression of rat AT1-AS The efficient expression of antisense AT1R mRNA is crucial for blocking AT1R and therefore in the present study, the expression of the rat AT1-AS was analyzed using semiquantitative RT-PCR. Total RNA was prepared from animal hearts, kidneys, and aorta 12 weeks after exposure to a high-salt diet. RT-PCR was carried out using primers specific to rat native AT1R cDNA (base pair 501) rather than transfected antisense cDNA or its mRNA. As shown in Figure 7, in the salt-induced, hypertensive animals (rAAV-GFP group), the AT1R mRNA level was significantly upregulated in the heart, kidney, and aorta compared with the normal control group. In contrast, rAAV-AT1-AS treatment markedly downregulated the transcription of AT1R in rats compared with the control groups, including both rAAV-GFP-treated and normal control rats. Furthermore, we reviewed the AT1R protein expression and found that AT1R was unregulated in the heart, kidney, and liver of the salt-induced, hypertensive rats; rAAV-AT1-AS treatment dramatically attenuated the effect to a lower level than the normal control (Figure 7). The results suggest that the rAAV-mediated partial AT1R antisense cDNA delivery was not only transcripted, but also efficiently blocked the transcription of AT1R mRNA in the main organs, therefore preventing the development of hypertension and protecting against hypertension-induced target organ injury.
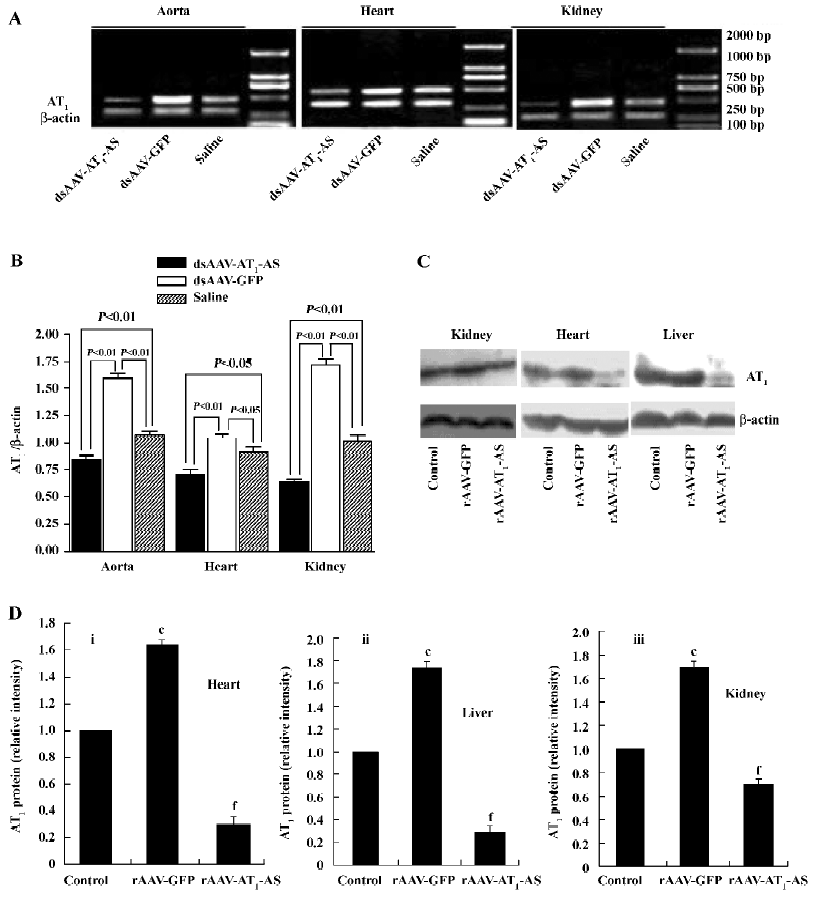
Discussion
The AAV has many advantages and properties compared with other vectors, such as retroviruses or adenovirus, including safety, the ability to infect both the dividing and non-dividing cells, and the lack of pathogenesis or inflammatory response. In the present study, therefore, we constructed the rAAV with antisense to AT1R. The rAAV vector has been shown to drive an efficient and prolonged transgene expression, either via persistent episomal concatamers[27,28] or by genomic integration[21,22].
It is well known that a high-salt diet is an important inducer for hypertension[29–31]. Scientists have found that high salt can induce the overexpression of AT1R in vascular smooth muscles both in vivo and in vitro[7,8], and subsequently enhance the re-absorption of water and salt; that is why a high-salt diet induces hypertension. Moreover, high salt can induce the overexpression of AT1 in the kidneys and promotes fibrosis and hypertrophy of the kidneys and heart[32-35]. The clinical applications of a new class of antihypertensive drug AT1 blockades resulted in efficient reduction, organ protection, and improved long-term prognosis[36,37], which provides further support for our hypothesis. Recently, Phillips et al exploited the AAV and retroviral vectors to mediate AT1-AS-induced hypotension in SHR and 2K1C rats[11,38–42], which suggests the possibility of the antisense AT1 gene to treat hypertension through systemic delivery. However, the hypotensive effect of rAAV-mediated antisense AT1 on high-salt diet-induced hypertension has not been documented.
In this study, we used rAAV, an efficient and rapid expression vector, to mediate partial AT1-AS (660 base pairs) delivery. The results showed that 1 bolus injection of rAAV-AT1-AS prevented a rise in blood pressure in high-salt diet-induced hypertensive rats in the long term, and this effect can come as early as 1 week after injection.
RT-PCR showed that systemic rAAV-AT1-AS delivery markedly reduced the transcription level of AT1 in the heart, aorta, and kidney of rats in the long term, which not only led to a sustained reduction in the systolic blood pressure, but also significantly decreased the urine levels of microalbumin and protected the kidneys from injury and fibrosis of glomerulus induced by hypertensive rats. A morphological analysis showed that the damage in the rAAV-GFP-treated rats also included tubular dilatation, loss of brush border in the proximal tubule, and glomerular sclerosis. Large areas of intense focal fibrosis in the cortex and the medulla vascular area, indicated by ECM staining, were observed in the control group, whereas AT1-AS gene delivery greatly attenuated all these renal damages and reduced the size and number of protein casts present in the tubules.
Previous studies have documented that high salt plays an important role in cardiovascular remodeling. The mechanisms through which high salt induces cardiovascular and renal remodeling may be direct biological effects[33–35]. Pachori et al administered the retroviral vector containing AT1R antisense in the neonatal rat, which resulted in a significant attenuation of cardiac hypertrophy despite its failure to normalize high blood pressure in renin-transgenic rats[43]. In our study, rAAV-AT1-AS treatment significantly attenuated cardiomyocyte diameter and fibrosis, as observed by reduced ECM staining, as well as homodynamic variable deterioration and a marked alleviation of diastolic and contractile dysfunction compared with the rAAV-GFP-treated animals. The results show that the long-term, stable expression of the AT1-AS treatment efficiently protects the heart and improves cardiac function in salt-induced, hypertensive rats.
In summary, this study shows that the delivery of rAAV-mediated partial antisense cDNA to the AT1R has the potential for chronically preventing hypertension in high-salt diet animals. The hypotensive effect was sustained and persisted for over 3 months in the high-salt diet rats, and protected the heart and kidney from damage. These findings suggest the feasibility of rAAV-AS for the gene therapy of hypertension and its complications. No adverse effects induced by rAAV injection were observed during the course of the study. The high level of expression from rAAV makes it attractive for human gene therapy. More experiments are needed to study the immunological aspects and the influence of the overexpression of the AT1-AS gene on diverse biological processes before this approach is utilized clinically in the therapy of hypertension and other cardiovascular and renal diseases.
References
- Folkow B. Critical review of studies on salt and hypertension. Clin Exp Hypertens A 1992;14:1-14.
- Haddy FJ, Pamnani MB. Role of dietary salt in hypertension. J Am Coll Nutr 1995;14:428-38.
- Muntzel M, Druecke T. A comprehensive review of the salt and blood pressure relationship. Am J Hypertens 1992;5:1-42.
- Weinberger MH. Sodium sensitivity and blood pressure. Curr Opin Nephrol Hypertens 1993;2:935-9.
- Reid I. The renin–angiotensin system: physiology, pathophysiology, and pharmacology. Advan Physiol Edu 1998;275:236-45.
- Alderman MH, Madhavan S, Ooi WL, Cohen H, Sealy JE, Laragh JH. l. Association of the renin-sodium profile with the risk of myocardial infarction in patients with hypertension. New Engl J Med 1991;324:1098-104.
- Nickenig G, Strehlow K, Roeling J, Zolk O, Knorr A, Böhm M. Salt induces vascular AT1 receptor overexpression in vitro and in vivo. Hypertension 1998;31:1272-7.
- Gonzalez M, Lobos L, Castillo F, Gastillo F, Gallequillos L, Lopez NC, Michea L. High-salt diet inhibits expression of angiotensin type 2 receptor in resistance arteries. Hypertension 2005;45:853-9.
- Phillips MI. Gene therapy for hypertension. Hypertension 2001;38:543-8.
- Iyer SN, Lu D, Katovich MJ, Raizada MK. Chronic control of high blood pressure in the spontaneously hypertensive rat by delivery of angiotensin type 1 receptor antisense. Proc Natl Acad Sci USA 1996;93:9960-5.
- Gyurko R, Wielbo D, Phillips MI. Antisense inhibition of AT1 receptor mRNA and angiotensinogen mRNA in the brain of spontaneously hypertensive rats reduces hypertension of neurogenic origin. Regul Pept 1993;49:167-74.
- Phillips MI, Wielbo D, Gyurko R. Antisense inhibition of hypertension: a new strategy for renin–angiotensin candidate genes. Kidney Int 1994;46:1554-6.
- Wielbo D, Sernia C, Gyurko R, Phillips MI. Antisense inhibition of hypertension in the spontaneously hypertensive rat. Hypertension 1995;25:314-9.
- Wang X, Sun Z, Cade R. Prolonged attenuation of cold-induced hypertension by adenoviral delivery of renin antisense. Kidney Int 2005;68:680-7.
- Peng JF, Kimura B, Fregly MJ, Phillips MI. Reduction of cold-induced hypertension by antisense oligodeoxynucleotides to angiotensinogen mRNA and AT1-receptor mRNA in brain and blood. Hypertension 1998;31:1317-23.
- Kimura B, Mohuczy D, Tang X, Phillips MI. Attenuation of hypertension and heart hypertrophy by adeno-associated virus delivering angiotensinogen antisense. Hypertension 2001;37:376-80.
- Wang H, Katovich MJ, Gelband CH. Sustained inhibition of angiotensin I-converting enzyme (ACE) expression and long-term antihypertensive action by virally mediated delivery of ACE antisense cDNA. Circ Res 1999;85:614-22.
- Muzyczka N, McLaughin S. Use of adeno-associated virus as a mammalian transduction vector. In: Gluzman Y, Hughes SH, editors. Current communications in molecular biology: viral vectors. Cold Spring Harbor, NY: Cold Spring Harbor Laboratory Press; 1988. p 39–44.
- Muzyczka N. Use of adeno-associated virus as a general transduction vector for mammalian cells. In: Current topics in microbiology and immunology. Berlin, Germany: Springer–Verlag; 1992. p 97–129.
- Samulski RJ, Zhu X, Xiao X, Brook JD, Housman DE, Estien N, et al. Targeted integration of adeno-associated virus (AAV) into human chromosome 19. EMBO J 1991;10:3941-50.
- Xiao X, Li J, Samulski RJ. Production of high-titer recombinant adeno-associated virus vectors in the absence of helper adenovirus. J Virol 1998;72:2224-32.
- Wang Z, Ma HI, Li J, Sun L, Zhang J, Xiao X. Rapid and highly efficient transduction by double-stranded adeno-associated virus vectors in vitro and in vivo. Gene Ther 2003;10:2105-11.
- Wang T, Li H, Zhao C, Chen C, Li J, Chao J, et al. Recombinant adeno-associated virus-mediated kallikrein gene therapy reduces hypertension and attenuates its cardiovascular injuries. Gene Ther 2004;11:1342-50.
- Auricchio A, Hildinger M, O’Connor E, Gao G, Wilson JM. Isolation of highly infectious and pure adeno-associated virus type 2 vectors with a single-step gravity-flow column. Hum Gene Ther 2001;12:71-6.
- Dobrzynski E, Wang C, Chao J, Chao L. Adrenomedullin gene delivery attenuates hypertension, cardiac remodeling, and renal injury in deoxycorticosterone acetate-salt hypertensive rats. Hypertension 2000;36:995-1001.
- Cindy W, Eric D, Julie C, Lee C. Adrenomedullin gene delivery attenuates renal damage and cardiac hypertrophy in Goldblatt hypertensive rats. Am J Physiol Ren Physiol 2001;280:F964-71.
- Yan Z, Zhang Y, Duan D, Englhardt J. From the cover: trans-splicing vectors expand the utility of adeno-associated virus for gene therapy. Proc Natl Acad Sci USA 2000;97:6716-21.
- Yang J, Zhou W, Zhang Y. Concatamerization of adeno-associated virus circular genomes occurs through intermolecular recombination. J Virol 1999;73:9468-77.
- Michael H. Alderman. Salt, blood pressure and health: a cautionary tale. Int J Epidemiol 2002;31:311-6.
- Intersalt. An international study of electrolyte excretion and blood pressure: results for 24 h urinary sodium and potassium excretion: Intersalt Cooperative Research Group. Brit Med J 1988;297:319-28.
- Mancilha-Carvalho JJ, Souza e Silva NA. The Yanomami Indians in the INTERSALT Study. Arq Bras Cardiol 2003;80:295-300.
- Sechi LA, Griffin CA, Giacchetti G, Valentin JP, Llorens-Cortes C, Corvol P, et al. Tissue-specific regulation of type 1 angiotensin II receptor mRNA levels in the rat. Hypertension 1996;28:403-8.
- Du Cailar G, Ribstein J, Mimran A. Dietary sodium and target organ damage in essential hypertension. Am J Hypertens 2002;15:222-9.
- de Wardener HE, MacGregor GA. Harmful effects of dietary salt in addition to hypertension. J Hum Hypertens 2002;16:213-23.
- Safar ME, Thuilliez C, Richard V, Benetos A. Pressure-independent contribution of sodium to large artery structure and function in hypertension. Cardiovasc Res 2000;46:269-76.
- Dahlof B, Devereux RB, Kjeldsen SE, Julius S, Beervers G, de Faire U, et al. Cardiovascular morbidity and mortality in the Losartan Intervention for endpoint reduction in hypertension study (LIFE): a randomised trial against atenolol. Lancet 2002;359:995-1003.
- Kjeldsen SE, Dahlof B, Devereux RB, Julius S, Aurup P, Edelman J, et al. Effects of losartan on cardiovascular morbidity and mortality in patients with isolated systolic hypertension and left ventricular hypertrophy: a Losartan Intervention for Endpoint Reduction (LIFE) substudy. JAMA 2002;288:1491-8.
- Galli SM, Phillips MI. Angiotensin II AT(1A) receptor antisense lowers blood pressure in acute 2-kidney, 1-clip hypertension. Hypertension 2001;38:674-8.
- Phillips MI, Mohuczy-Dominiak D, Coffey M. Prolonged reduction of high blood pressure with an in vivo, nonpathogenic, adeno-associated viral vector delivery of AT1-R mRNA antisense. Hypertension 1997;29:374-80.
- Phillips MI. Antisense inhibition and adeno-associated viral vector delivery for reducing hypertension. Hypertension 1997;29:177-87.
- Katovich MJ, Gelband CH, Reaves P. Reversal of hypertension by angiotensin II type 1 receptor antisense gene therapy in the adult SHR. Am J Physiol 1999;277:H1260-4.
- Reaves PY, Beck CR, Wang HW. Endothelial-independent prevention of high blood pressure in L-NAME-treated rats by angiotensin II type I receptor antisense gene therapy. Exp Physiol 2003;88:467-73.
- Pachori AS, Numan MT, Ferrario CM, Diz DM, Raizada MK, Katovich MJ. Blood pressure-independent attenuation of cardiac hypertrophy by AT(1)R-AS gene therapy. Hypertension 2002;39:969-75.