Interaction between hydrogen sulfide/cystathionine γ-lyase and carbon monoxide/heme oxygenase pathways in aortic smooth muscle cells1
Introduction
In the late 1980s, nitric oxide (NO) was first demonstrated as an endogenous gasotransmitter[1
However, gasotransmitters in vascular tissues might not exist independently, whereas they might have interactions[15]. NO was found to be derived from vascular endothelial cells, H2S is endogenously generated from vascular smooth muscle cells, while HO exists in both vascular endothelial cells and smooth muscle cells. This suggests that NO, CO and H2S might represent the most rapid communicative pathway between vascular endothelial cells and smooth muscle cells, being involved in regulating the biological actions. Vascular endothelial cells and smooth muscle cells are the main place for the production of gasotransmitters. At the same time, they are the target cells of gasotransmitters. The above facts provide the basis for the likelihood of interactions among gasotransmitters. Our previous study demonstrated that exogenous supplements of H2S could alleviate hypoxia-induced elevation of pulmonary arterial pressure. At the same time, H2S might impact the increased CO production in pulmonary arteries on a chronic hypoxic animal model. However, the above results were derived from hypoxic pulmonary hypertensive rats; whether there are any impacts of H2S on the CO pathway and vice versa in aortic smooth muscle cells (ASMC) under physiological conditions and its mechanisms remains unclear. Therefore, the present study was undertaken to examine the possible interaction between H2S/CSE and CO/HO pathways in ASMC.
Materials and methods
Smooth muscle cell culture Male Sprague-Dawley rats, weighing 200–220 g, were killed by anesthetic overdose (intraperitoneal injection of pentobarbital sodium). The thoracic aorta were stripped of adventitia and removed to culture media sterilely. The ASMCs were isolated and cultured according to the method used by Hirata et al[16]. Briefly, cells were maintained in DMEM containing 5 mmol/L glucose, 10% FBS, and antibiotics in a CO2 incubator at 37 oC. The subcultures of ASMC from passage 4 were used in the experiments and cells were cultured for 24 h in serum-free DMEM before each experiment. The cells were divided into 4 groups according to different treatments, and each group included at least 6 wells. (1) the control group, where cells were continuously cultured in serum-free DMEM; (2) the zinc protoporphyrin (ZnPP, an inhibitor of HO) group, where 20 mmol/L ZnPP was added; (3) the propargylglycine (PPG, an inhibitor of CSE) groups, where 2 mmol/L, 4 mmol/L and 10 mmol/L of PPG were added, respectively; and (4) the sodium hydrosulfide (NaHS, a H2S donor) groups, where 1×10-5 mol/L, 1×10-4 mol/L and 1×10-3 mol/L of NaHS were added, respectively. Each of the above groups was further divided into 6 h, 12 h, 18 h and 24 h subgroups. ZnPP (Sigma, St Louis, MO,USA) was dissolved in 50 mmol/L sodium carbonate. PPG (Sigma, St Louis, MO, USA) and NaHS (Sigma, St Louis, MO, USA) were dissolved in sterile water.
Measurement of carboxyhemoglobin (HbCO) in conditioned medium To examine the relative amount of CO being released from the ASMC into the medium, hemoglobin (50 µmol/L) was added to the cells during the last hour of incubation, and HbCO was measured by a spectrophotometric method[17]. Medium 0.5 mL was mixed with 1 mL of the hemoglobin solution, which was derived from a mixture of 0.25 mL of fresh-packed erythrocytes from rats and 50 mL of 0.25 mol/L ammonia solution. Then, 0.1 mL of the sodium dithionite was added. The difference of absorbance at 568 and 581 nm was read via the double-wavelength spectrophotometer. Both the wavelengths were selected according to the absorbance spectrum of HbCO and oxygenated hemoglobin (HbO2), at which the difference of absorbance of HbCO was largest and the difference of absorbance of HbO2 was 0. The percentage of HbCO was then calculated from a standard curve derived by mixing different proportions of 2 hemoglobin solutions containing 100% HbCO and 100% HbO2. We obtained these solutions by gassing hemoglobin solutions with pure oxygen and CO, respectively. For our spectrophotometer, the following relationship was obtained from the standard curve: %HBCO=[(OD568- OD581)-0.03858]/0.0498.
Measurement of H2S in conditioned medium H2S was measured with a sulfide sensitive electrode (Model 9616; Orion Research; Beverly, MA, USA) as described previously[18]. In brief, sulfide antioxidant buffer was added into standards or samples at a ration of 1:1 and then stirred thoroughly. Electrodes were rinsed in distilled water, blotted dry and placed into standards and samples. When a stable reading was displayed, the millivolt value was recorded. The H2S concentration was calculated against the calibration curve of the standard H2S solution.
Western blotting Cultured cells (3×106) were harvested and lysed in a lysis buffer (0.5 mol/L EDTA, 1 mol/L Tris-HCl, pH 7.4, 0.3 mol/L sucrose, 1 µg/mL antipain hydro-chloride, 1 mmol/L benzamidine hydrochloride hydrate, 1 µg/mL leupeptin hemisulfate, 1 mmol/L 1,10-phenanthroline monohydrate, 1 µmol/L pepstatin A, 0.1 mmol/L phenyl-methylsulfonyl fluoride and 1 mmol/L iodoacetamide). The extracts were clarified by centrifugation at 14 000×g for 15 min at 4 oC. SDS-PAGE and Western blot analysis were performed according to experimental protocol. The primary antibody dilutions were as follows: 1:2000 for HO-1 (Sigma, St Louis, MO, USA), 1:50 for CSE and 1:1000 for β-actin (TBD, Tianjin, China). HRP-conjugated secondary antibody was used at 1:5000. The immunoreactions were visualized by electrochemiluminescence (ECL) and exposed to X-ray film (Kodak Scientific Imaging film, X-Omat Blue XB-1, USA). Membranes were stripped by incubation in a buffer containing 100 mmol/L β-mercaptoethanol, 2% SDS, and 62.5 mmol/L Tris-HCl, pH 6.8.
Statistical analysis The results were expressed as Mean±SD. ANOVA was used to compare the mean values, including HbCO level in the cultured ASMC medium and HO-1 expression by the ASMC in the various groups. Post-hoc analysis was then used to compare the data between the different groups. Independent t-test was used to estimate the differences in H2S content in the cultured ASMC medium and CSE expression by the ASMC between the control group and the ZnPP group. P<0.05 was considered statistically significant.
Results
Impact of endogenous CO on the H2S/CSE pathway To examine the impact of endogenous CO on H2S release by the ASMCs in the medium, the ASMCs were treated with ZnPP at a concentration of 20 µmol/L. After ZnPP treatment for 6 h, 12 h,18 h and 24 h, the H2S content in the medium markedly increased by 11.81%, 18.79%, 41.58% and 12.19%, respec-tively, as compared with that in the medium without ZnPP treatment (all P<0.01) as seen in Table 1. The results also showed that the peak time point of the augmentation was at 18 h after the treatment of ZnPP.
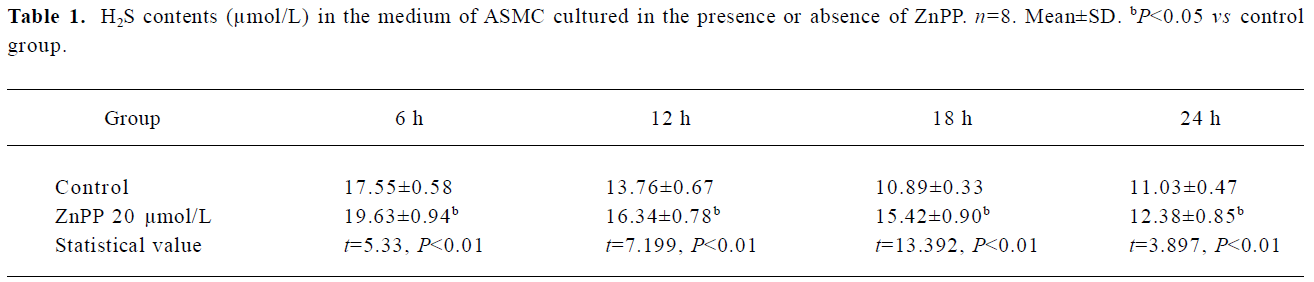
Full table
After the treatment of ZnPP at a concentration of 20 µmol/L with cultured ASMC for 6 h, 12 h,18 h, and 24 h, CSE expression by ASMC markedly increased by 35.73%, 31.94%, 58.22% and 16.78%, respectively, compared with the controls (all P<0.01). The peak time point of the augmentation was also at 18 h after the the ZnPP treatment (Figure 1).
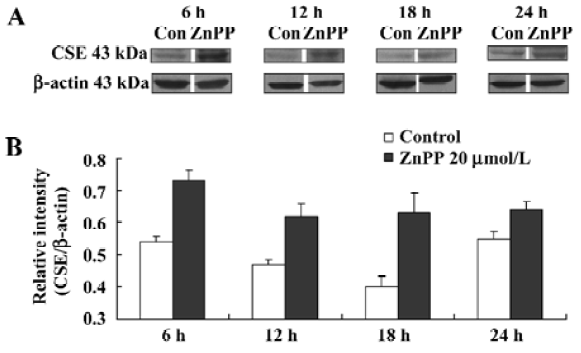
Impact of endogenous H2S on the CO/HO pathway To examine the impact of endogenous H2S on CO release by medium of cultured ASMC, the ASMCs were treated with PPG at a concentration of 2 mmol/L, 4 mmol/L and 10 mmol/L, respectively. After PPG treatment at each concentration for 6 h and 12 h, there were no significant changes in CO release by cultured ASMC as seen in Table 2 (P>0.05). The CO release started to be augmented by 4.02% following the 24 h treatment with PPG at 2 mmol/L, but was clearly augmented by 7.11% and 7.78% following 18 h and 24 h treatment with PPG at 4 mmol/L, respectively (P<0.01). Also, in the presence of PPG at 10 mmol/L, CO release markedly increased by 5.38% and 15.57% compared with the controls at 18 h and 24 h, respectively (all P<0.01; Table 2).
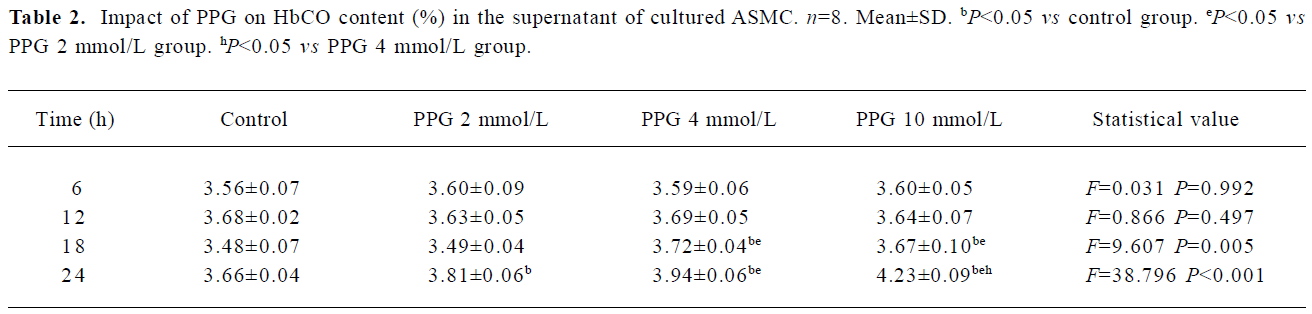
Full table
After PPG treatment at each concentration for 6 h, there were no significant changes in HO-1 expression by cultured ASMC (P>0.05). The HO-1 expression by cultured ASMC started to be strengthened by 34.95% following 24 h treatment with PPG at a concentration of 2 mmol/L, but was evidently strengthened by 169.19% and 54.42% after 18 h and 24 h treatment with PPG at 4 mmol/L, respectively(P<0.01). In the presence of PPG at 10 mmol/L, HO-1 expression by cultured ASMC markedly increased by 60.29%, 102.31% and 243.36% compared with the controls at 12, 18 h and 24 h, respectively (all P<0.01). The results also showed that PPG increased HO-1 expression by cultured ASMC at 24 h in a dose-dependent manner (P<0.01; Figure 2).
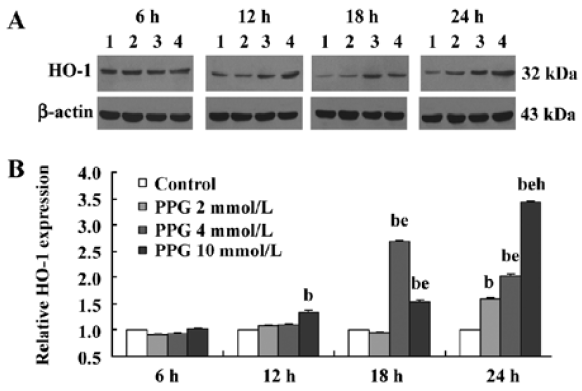
Impact of H2S donor on the CO/HO pathway To examine the impact of H2S on CO release by cultured ASMC, the ASMCs were treated with NaHS, a H2S donor, at a concentration of 1×10-5 mol/L, 1×10-4 mol/L, and 1×10-3 mol/L, respectively (Table 3). After treatment for 6 h, NaHS at 1×10-4 mol/L and 1×10-3 mol/L decreased HbCO content in the supernatant of cultured ASMC by 3% and 5.09% (all P<0.05), respectively. After treatment for 12 h, NaHS at each concentration decreased HbCO content in the supernatant of cultured ASMC by 2.74%, 5.47%, and 8% (all P<0.05) in a dose-dependent manner. After treatment for 18 h and 24 h, NaHS at 1×10-5 mol/L and 1×10-4 mol/L did not change the HbCO content in the supernatant (all P>0.05), while NaHS at 1×10-3 mol/L reduced the HbCO content in the supernatant significantly by 5.37% and 8.22% (all P<0.05), respectively.
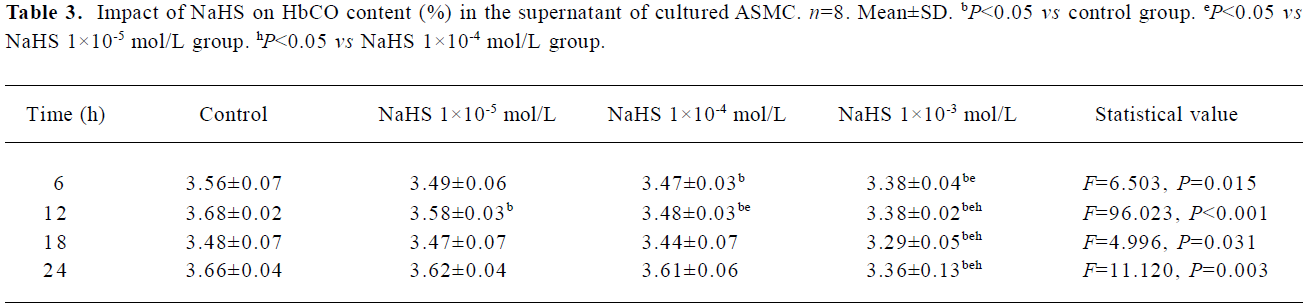
Full table
After NaHS treatment at each concentration for 6 h and 12 h, HO-1 expressions by cultured ASMC decreased in a dose-dependent manner (all P<0.05). The results also showed that NaHS at 1×10-3 mol/L inhibited HO-1 expression by cultured ASMC at all time points of the treatments, while NaHS at 1×10-5 mol/L and 1×10-4 mol/L inhibited HO-1 expression by cultured ASMC at 6 h and 12 h of the treatment (Figure 3).
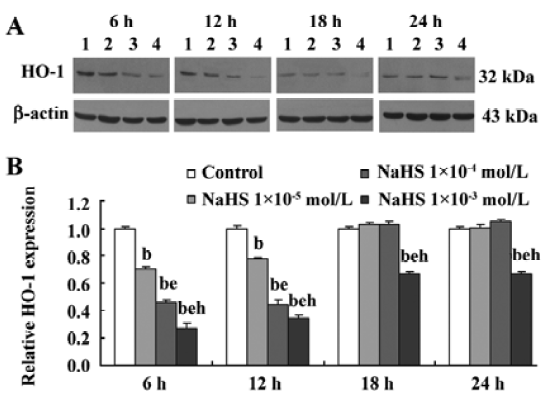
Discussion
Hydrogen sulfide (H2S) has been recognized as a toxic gas in water pollution and industrial air pollution with a strong odor for long time. More interestingly, Hosoki et al[10] found that H2S could be produced in the portal vein, thoracic aorta, tail artery, mesenteric artery and pulmonary artery. The vascular smooth muscle was relaxed by the treatment of H2S in a dose-dependent manner, including intact endothelia and removed endothelia, and in synergy with NO. Endogenous H2S in rat vascular tissues, as a vascular relaxant factor, could maintain the basal blood pressure balance under physiological conditions[9]. It could also suppress the proliferation of cultured vascular smooth muscle cells (vascular smooth muscle cells) through the mitogen-activated protein kinase pathway in a dose-dependant manner in vitro[11]. Based on its endogenous metabolism and physiological functions, H2S is well positioned in the novel family of endogenous gaseous transmitters. More and more evidence is proving that H2S might be the third endogenous signaling gasotransmitter besides NO and CO[7]. CO acts as a second gasotransmitter that shares some of the physiochemical properties of NO[15]. Like NO, CO can bind to the iron atom of the heme moiety associated with soluble guanylyl cyclase, thereby activating the enzyme and increasing intracellular cGMP produc-tion. CO is produced endogenously by various cell types as a byproduct of heme catabolism, in which HO catalyzes the degradation of heme into equimolar amounts of biliverdin, iron and CO[5].
Recently, the rapid development of systems biology makes us aware that only a single molecule or its pathway cannot regulate the cardiovascular functions independently[19]. It was discovered that H2S is formed in VSMC, while CO is generated mainly in VSMC, but also in vascular endothelial cells, acting on vascular cells in an autocrine/paracrine manner. Previous studies have also demonstrated that gasotransmitters always interact. For instance, previous studies have indicated that endogenous CO/HO and NO/nitric oxide synthase (NOS) interacted with each other, as did NO/NOS and H2S/CSE pathways, therefore playing important roles in regulating the homeostasis of the cardiovascular system[20,21]. However, how CO/HO and H2S/CSE pathways interact is still not clear.
For the purpose of understanding if there are any interactions between CO on the H2S pathway in ASMC under physiological conditions, we performed the above studies culturing ASMC treated with the CO/HO inhibitor, H2S/CSE inhibitors and H2S donor, respectively. We noticed that there was an impact of endogenous CO on the H2S/CSE pathway in ASMC. We observed that after the ASMC were treated with ZnPP, an inhibitor of HO[22], the H2S content in the medium markedly increased compared to the controls. The results demonstrated that endogenous CO could down-regulate H2S production under physiological conditions. The mechanisms, however, were not fully understood. As we know, CSE was the main enzyme in ASMC catalyzing the endogenous production of H2S. Therefore, we speculated that the induced ASMC CSE expression by ZnPP treatment might be involved in the mechanism by which endogenous CO regulated the H2S/CSE pathway. In our study, we found that CSE expression by ASMC treated with ZnPP markedly increased compared with that untreated with ZnPP. How-ever, further studies should be conducted to understand the impact of endogenous CO on the CSE gene expression and modulations.
To understand if there are any impacts of H2S on the CO pathway in ASMC under physiological condition, we performed the present study and found that after treatment with PPG[23], an inhibitor of CSE in cultured ASMC, CO release by ASMC was augmented. Moreover, NaHS, a H2S donor, decreased HbCO content in the supernatant of cultured ASMC. The above results indicated that H2S could markedly inhibit CO release by ASMC under physiological conditions. To examine the possible mechanisms by which H2S regulated endogenous CO production, we employed Western blot to detect HO-1 expression by ASMC. Our data showed that PPG strengthened the HO-1 expression by cultured ASMC, while NaHS inhibited the HO-1 expression by cultured ASMC. The results revealed that the mechanisms by which H2S regulated endogenous CO production involved at least the inhibition of HO-1 expression by cultured ASMC. However, further studies dealing with the influence of endogenous H2S on HO-1 transcription in addition to its translation should be done.
The interaction between CO and H2S may have potential physiological significance. Both CO and H2S were produced in VSMC and have distinct characteristics, including fast production, rapid diffusion, extensive action and a shorter life time. They also share similar biological functions. For vascular regulation, both were demonstrated to relax the vessels and inhibit the VSMC proliferation. However, they are different in many aspects, including their signaling pathways. The coordinated regulation of these species suggests that they all are used in cell signaling. These reactions establish “cross-talk” between 2 VSMC-derived gaso-transmitters. Such adaptive mechanisms are thought to be of evolutionary importance[24]. However, investigations on the interactions among gasotransmitters and other vasoactive substances need to be further conducted.
References
- Koshland DE. The molecule of the year. Science 1992;258:1861.
- Ignarro LJ. Biosynthesis and metabolism of endothelium-derived nitric oxide. Annu Rev Toxicol 1990;30:535-60.
- Li SJ, Sun NL. Regulation of intracellular Ca2+ and calcineurin by NO/PKG in proliferation of vascular smooth muscle cells. Acta Pharamcol Sin 2005;26:323-8.
- Wang YY, Tang ZY, Dong M, Liu XY, Peng SQ. Inhibition of platelet aggregation by polyaspartoyl L-arginine and its mecha-nism. Acta Pharmacol Sin 2004;25:469-73.
- Maines MD. The heme oxygenase system: a regulator of second messenger gases. Annu Rev Pharmacol Toxicol 1997;37:517-54.
- Guidotti TL. Hydrogen sulfide. Occup Med 1996;46:367-71.
- Du JB, Chen XB, Geng B, Jiang HF, Tang CS. Hydrogen sulfide as a messenger molecule in cardiovascular system. Beijing Da Xue Xue Bao 2002;34:187. Chinese..
- Stipanuk MH, Beck PW. Characterization of the enzymiccapacity for cysteine desulphhydration in liver and kidney of the rat. Biochem J 1982;206:267-77.
- Kimura H, Nagai Y, Umemura K, Kimura Y. Physiological roles of hydrogen sulfide:synaptic modulation, neuroprotection, and smooth muscle relaxation. Antioxid Redox Signal 2005;7:795-803.
- Hosoki R, Matsuki N, Kimura H. The possible role of hydro-gensulfide as an endogenous smooth muscle relaxant in synergy with nitric oxide. Biochem Biophys Res Commun 1997;237:527-31.
- Du J, Cheung Y, Bin G, Jiang H, Chen X, et al. The possible role of hydrogen sulfide as a smooth muscle cell proliferation inhibitor in rat cultured cells. Heart Vessels 2004;19:75-80.
- Yang G, Sun X, Wang R. Hydrogen sulfide-induced apoptosis of human aorta smooth muscle cells via the activation of mitogen-activated protein kinases and caspase-3. FASEB J 2004;18:1782-4.
- Geng B, Yang J, Qi Y, Zhao J, Pang Y, Du J, et al. H2S generated by heart in rat and its effect on cardiac function. Biochem Biophys Res Commun 2004;313:362-8.
- Zhao W, Zhang J, Lu Y, Wang R. The vasorelaxant effect of H2S as a novel endogenous gaseous KATP channel opener. EMBO J 2001;20:6008-16.
- Hartsfield CL. Cross talk between carbon monoxide and nitric oxide. Antioxid Redox Signal 2002;4:301-7.
- Hirata Y, Taragi Y, Takata S. CGRP receptor in cultured vascular smooth muscle and endothelial cells. Biochem Biophys Res Commun 1988;151:1113-8.
- Shi Y, Du JB, Gong LM, Zeng CM, Tang XY, Tang CS. The regulating effect of heme oxygenase/carbon monoxide on hypoxic pulmonary vascular structural remodeling. Biochem Biophys Res Commun 2003;306:523-9.
- Chen YH, Yao WZ, Geng B, Ding YL, Lu M, Zhao MW, et al. Endogenous hydrogen sulfide in patients with COPD. Chest 2005;128:3205-11.
- Weston AD, Hood L. Systems biology, proteomics, and the future of health care: toward predictive, preventative, and personalized medicine. J Proteome Res 2004;3:179-96.
- Shi Y, Du JB, Guo ZL, Zeng CM, Tang CS., Yun S, et al. Interaction between endogenous nitric oxide and carbon monoxide in the pathogenesis of hypoxic pulmonary hypertension. Chin Sci Bull 2003;48:86-90.
- Zhang QY, Du JB, Shi L, Zhang CY, Yan H, Tang CS. Interaction between endogenous nitric oxide and hydrogen sulfide in pathogenesis of hypoxic pulmonary hypertension. Beijing Da Xue Xue Bao 2004;36:52-6. Chinese..
- Zhang M, Zhang BH, Chen L, An W. Overexpression of heme oxygenase-1 protects smooth muscle cells against oxidative injury and inhibits cell proliferation. Cell Res 2002;12:123-32.
- Yan H, Du JB, Tang CS. The possible role of hydrogen sulfide on the pathogenesis of spontaneous hypertension in rats. Biochem Biophys Res Commun 2004;313:22-7.
- Pryor WA, Houk KN, Foote CS, Fukuto JM, Ignarro LJ, Squadrito GL, et al. Free radical biology and medicine: it’s a gas, man! Am J Physiol Regul Integr Comp Physiol 2006;291:R491-511.