Intrathecal administration of Cav3.2 and Cav3.3 antisense oligonucleotide reverses tactile allodynia and thermal hyperalgesia in rats following chronic compression of dorsal root of ganglion
Introduction
Peripheral or central nerve injury results in neuropathic pain, manifesting tactile allodynia and hyperalgesia[1]. While many kinds of ionic conductance contribute to the development of neuropathic pain, voltage dependent calcium channels (VDCC) are unique in triggering physiological responses such as neurotransmitter release and calcium-dependent gene expression which have a key role in nociceptive message transmitting[2]. Currents arising from VDCC are subdivided into 2 major classes based on the membrane potential at which they become activated: high-voltage activated (HVA) or sustained currents which are further divided into L-, P-, Q-, N- and R-subtypes and low-voltage activated or transient (T-type) calcium currents which are further divided into Cav3.1, Cav3.2, and Cav3.3[3]. The HVA channels are activated with strong membrane depolarizations and are important for shaping action potentials and regulating transmitter release while T-type calcium channels are active at resting membrane potentials and play a crucial role in the regulation of cellular excitability[4,5].
Mibefradil, a selective antagonist of T-type channels, has an antinociceptive effect in neuropathic pain[6]. T-type calcium channels are also involved in long-term potentiation in synaptic transmissions between nociceptive primary afferents and superficial laminae neurons of the dorsal horn[7]. A pronociceptive role of these channels was suggested using weakly selective T-type channel antagonists in animals with neuropathic pain[8]. However, these blockers have no selectivity to the subtypes of T-type channels such as Cav3.1, Cav3.2 or Cav3.3; as well as this, they also can block the HVA if the doses are sufficient[9]. The role of those subtypes in the spinal cord in neuropathic pain has not been established. In the present study, we inhibit mRNA expression of the Cav3.1, Cav3.2, and Cav3.3 by intrathecal administration of antisense oligonucleotide, as well as observe the changes of the tactile allodynia and thermal hyperalgesia in the chronic compression of dorsal root ganglion (CCD) rats.
Materials and methods
Animals Adult male Sprague–Dawley (SD) rats weighing 250–280 g were housed in groups of 3–4 in plastic cages with soft bedding and free access to food and water under a 12:12 h day/night cycle. The rats were kept 5–7 d under these conditions before the experiments. The rats were supplied by the Laboratory Animal Center of Xuzhou Medical College (Xuzhou, China). All the experiments were approved by the Animal Care and Use Committee at the College and were in accordance with the guidelines for the Care and Use of Laboratory Animals.
Drugs Antisense phosphorodiester oligonucleotide (ODN) were designed based on the rat Cav3 sequence (Genbank N
Cav3.1 antisense oligonucleotide (Cav3.1–AS): CGAGA-CCCATTGGCATCCCT; Cav3.1 mismatch oligonucleotide (Cav3.1 mismatch): GCACGACCTATGCGACTCTC; Cav3.2 antisense oligonucleotide (Cav3.2–AS): CCACCTTCTACG-CCAGCGG; Cav3.2 mismatch oligonucleotide (Cav3.2 mismatch): CACTCTCTCACCAGGCGGC; Cav3.3 antisense oligonucleotide (Cav3.3–AS): GCTGAGGCGGCTTGTGTTT; and Cav3.3 mismatch oligonucleotide (Cav3.3 mismatch): CGTGAGCTGGCTGTGTTGT.A blast search revealed that these mismatch ODN were not complementary to any registered nucleotide sequences.
Surgical preparation
Implantation of intrathecal catheters (IT) The catheter was implanted into the rats according to the procedure originally described for chronic IT implant surgery[10]. To place the IT catheter, the rats were anesthetized with 4% isoflurane in a room air/oxygen mixture (1:1) and the back of the head and neck was shaved. The animals were then placed in a stereotaxic head holder with the head flexed forward. Anesthesia was maintained with 2% isoflurane delivered by a mask. A midline incision was made on the back of the neck. The muscle was freed at the attachment to the skull exposing the cisternal membrane. The membrane was opened with a stab blade, and an 7.5 cm polyethylene catheter was then inserted through the cisternal opening and passed carefully and caudally into the IT space at the rostral edge of the lumbar enlargement. The end of the catheter was tunneled through the sc space over the frontal bones, flushed with 10 uL saline, and then plugged with a short length of wire. The animals were allowed to recover from the implantation surgery 3–5 d prior to any experimentation and monitored daily after surgery for signs of motor deficiency.
Animal model of neuropathic pain[11] The rats were anesthetized with pentobarbital sodium (40 mg/kg, ip, supplemented as necessary). On the left side, the paraspinal muscles were separated from the mammillary process and the transverse process and the intervertebral foramina of L4 and L5 were exposed. An L-shaped stainless steel rod, 3.5 mm in length and 0.7 mm in diameter, was inserted approximately 3.5 mm into the intervetebral foramen at L4, and again at L5, at a rostral direction at an angle of 30–40 degrees to the dorsal middle line and 10 to 15 degrees below the vertebral horizontal. The purpose of compressing 2 DRG instead of 1 was to increase the number of compressed neurons innervating the plantar surface of the hind paw. As the rod was moved over the ganglion, the ipsilateral hind leg muscles typically exhibited 1 or 2 slight twitches. After the rod was in place, the muscle and skin layers were sutured.
Evaluation of thermal hyperalgesia and tactile allodynia
Thermal hyperalgesia The Hargreaves[12] test was used to determine the presence of the thermal hyperalgesia by measuring paw thermal withdrawal latency (TWL) to heat stimulation. The rat was placed on the surface of a 2-mm thick glass plate covered with a plastic chamber (20 cm×20 cm×25 cm), the latency of paw withdrawal responding to heat stimuli was measured with a radiant thermal stimulator (BME410A, Institute of Biological medicine, Academy of Medical Science, Tianjin, China). A heat source was focused on a portion of the hind paw, which was flushed against the glass, and a radiant thermal stimulus was delivered to that site. The stimulus shut off automatically when the hind paw moved, a 25 s cutoff was imposed on the stimulus’ duration to prevent tissue damage. The intensity of the heat stimulus was constant throughout all the experiments. Five stimuli were imposed to the same site repeatedly, and the mean TWL was obtained from the latter 3 stimuli. This value was taken as the steady state in the TWL values.
Tactile allodynia Mechanical withdrawal threshold (MWT) was measured by applying a von Frey hair (Stoelting, Wood Dale, IL, USA) to the hind paw until a positive sign of pain behavior was elicited[13]. The paradigm for assessing the threshold was as follows: the area tested was the mid-plantar paw in the area of the sciatic nerve distribution, avoiding the footpads. The von Frey filaments with logarithmically incremental stiffness (0.4–15.1 g) were applied serially to the paw by the up–down method. The hairs were presented in ascending order of strength, perpendicular to the plantar surface with sufficient force to cause slight bending against the paw and held for 6–8 s. A positive response was noted if the paw was sharply withdrawn. Flinching immediately upon removal of the hair was also considered a positive response. The 15.1 g hair was selected as the upper limit cutoff for testing. If there was no response at 15.1 g pressure, the animals were assigned this cutoff value. A bending force which can evoke 50% of the paw withdrawal occurrence was set as the MWT.
Drug administration and experimental paradigm Five days after IT catheter placement, the L4 and L5 DRG CCD was performed. Male SD rats were divided into 8 groups: CCD+NS group (CCD), Sham+NS group (sham), CCD+Cav3.1–AS group (Cav3.1–AS), CCD+Cav3.1 mismatch group (Cav3.1 mismatch); CCD+Cav3.2–AS group (Cav3.2–AS), CCD+Cav3.2 mismatch group (Cav3.2 mismatch); CCD+Cav3.3–AS group (Cav3.3–AS), and the CCD+Cav3.3 mismatch group (Cav3.3 mismatch). After the CCD model was established, NS (10 µL) or Cav3.1 antisense oligonucleotide, Cav3.1 mismatch oligonucleotide, Cav3.2 antisense oligonucleotide, Cav3.2 mismatch oligonucleotide, Cav3.3 antisense oligonucleotide, and Cav3.3 mismatch oligonucleotide 12.5 µg in 10 µL NS were given IT twice per day from the first day to the fourth day after operation. The MWT and TWL of the rats in every group (n=8) were measured before operation and every day from the first day to the fifteenth day after operation.
Western blotting Drugs or NS was given IT twice per day from the first day to the fourth day after operation. On the fifth day, the lumbosacral spinal cords of the rats in every group (n=6) were extracted and stored in liquid nitrogen. Tissue samples were homogenized in lysis buffer A (in mmol/L): Tris-HCl 20.0, Na3VO4 1.0, MgCl2 1.5, KCl 10.0, edetic acid (EDTA) 0.1, egtazic acid (EGTA) 0.1, phenylmethylsulfonyl fluoride (PMSF) 0.5 and 0.02% protease inhibitor cocktail (pH 7.9). After the addition of 90 μL NP-40 (10%), the homogenates were vortexed for 30 s and then centrifuged at 800×g for 15 min at 4 oC. Then the supernatants were centrifuged at 10 000×g for 1 h at 4 oC. Centrifugations were homogenized in lysis buffer B (in mmol/L): Tris-HCl 20.0, Na3VO4 0.03, MgCl2 2.0, KCl 10.0, EDTA 2.0, EGTA 2.0, PMSF 2.0, 0.1% TritonX-100, NaF 5.0 and 0.02% protease inhibitor cocktail. The homogenates were centrifuged at 10 000×g for 1 h at 4 oC. The supernatants were used for Western blot analysis as membranes proteins. Protein concentrations were determined using the Bradford method and the protein samples were stored at -80 oC. Ptoyrin samples were dissolved in 4×sample buffer (in mmol/L: Tris-HCl 250.0, sucrose 200.0, dithiothreitol (DTT) 300.0, 0.01% Coomassie brilliant blue-G, and 8% SDS, pH 6.8), and denatured at 95 oC for 5 min, then the equivalent amounts of proteins were separated by using 7.5% SDS-PAGE and transferred onto a nitrocellulose membrane. The membranes were incubated overnight at 4 oC with the following primary antibodies: rabbit anti-Cav3.1 antibody or Cav3.2 antibody or Cav3.3 antibody (Santa Cruz Biotechnology, California, USA). The membranes were extensively washed with Tris-buffered saline Tween-20 and incubated for 2 h with the secondary antibody with peroxidase-conjugated affinipure goat anti-rabbit IgG at room temperature. The immune complexes were detected with diaminobenzidine (DAB)assay kit (Zhongshan Biotechnology Co, Beijing, China).The scanned images were imported into Adobe Photoshop software (Adobe, California, USA).Scanning densitometry was used for semiquantitative analysis of the data.
Data analysis and statistics Data are expressed as mean±SD. The results were analyzed by analysis of the repeated-measures ANOVA followed by post-hoc comparison test to compare data obtained from the experiments. Values of P<0.05 were considered statistically significant.
Results
Mechanical allodynia and thermal hyperalgesia Mechanic tactile allodynia and thermal hyperalgesia were evident 1 d after CCD lasting at least 15 d. NS, Cav3.1–AS or every mismatch did not modify mechanical allodynia or thermal hyperalgesia. However, IT administration of Cav3.2–AS and Cav3.3–AS induced a significant increase in MWT and TWL compared to their respective mismatch group or NS group (Figures 1,2,3,4,5,6).
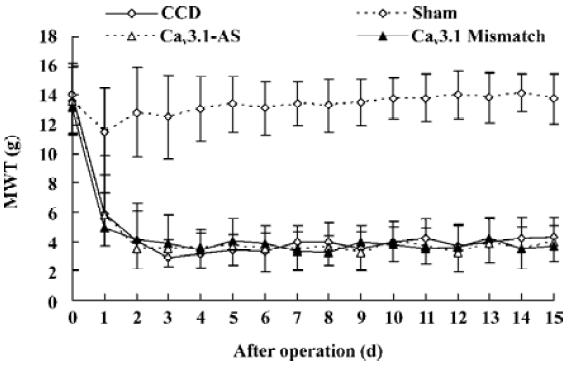
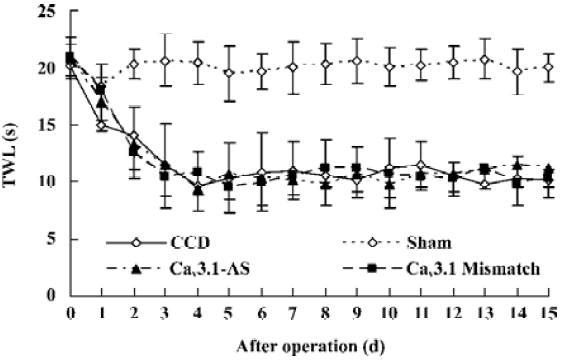
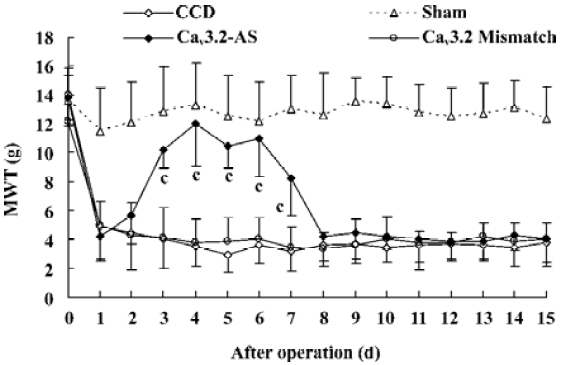
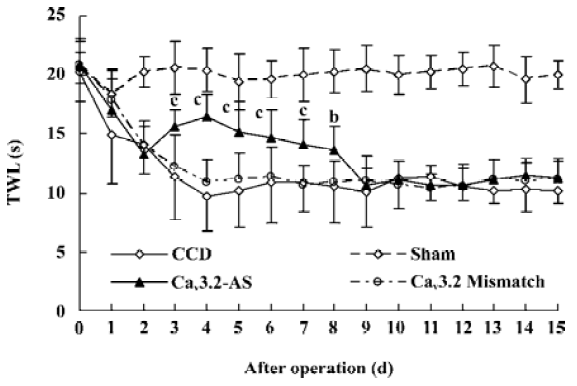
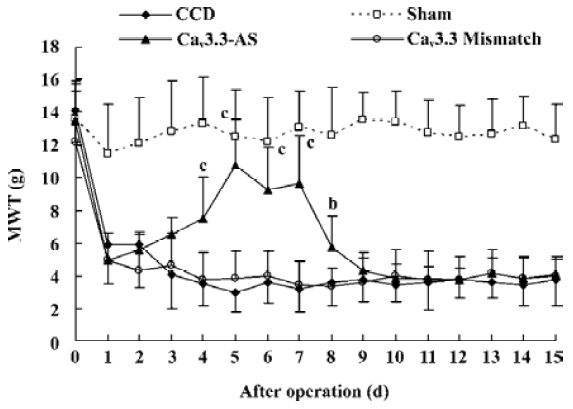
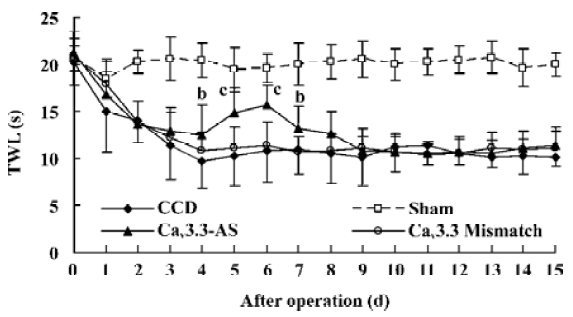
Protein expression of Cav3.2 and Cav3.3 after IT administration of antisense oligonucleotide, respectively We did not detect the expression of Cav3.1 protein in the spinal cord of CCD or the sham rats by Western blotting. However, we detected the expression of Cav3.2 and Cav3.3 proteins in the sham rats, and we found that the expression of Cav3.2 and Cav3.3 protein increased in CCD pathogenesis. Intrathecal administration of Cav3.2 and Cav3.3 antisense oligonucleotide can inhibit their protein expression respectively, but intrathecal administration of the mismatch oligonucleotide has no effect on the expression of their protein compared to the CCD group (Figures 7,8).
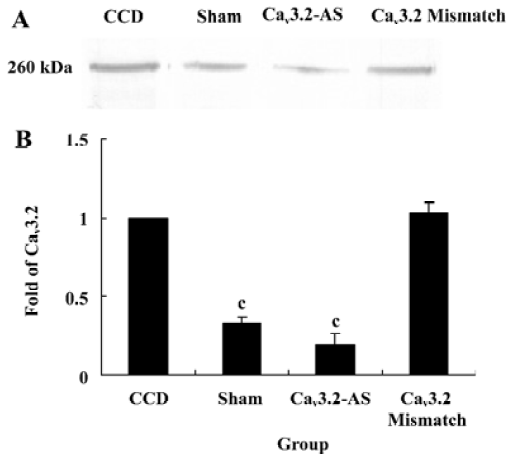
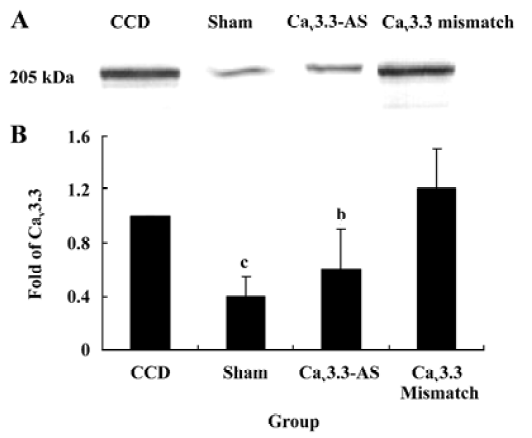
Discussion
Hyperexcitability of nociceptive neurons is a hallmark of neuropathic and inflammatory pain which can result in conditions of spontaneous pain, allodynia and hyperalgesia. T-type calcium channels regulate cellular excitability and rhythmic activity, and they contribute to pathophysiological conditions linked to neuronal hyperexcitability[14]. Recent data show that T-channels may play important roles in modulating peripheral nociceptive pathways[15]. Systemic administration of mibefradil in clinically relevant doses causes mechanical and thermal antinociception in adult rats, and this suggests that the antinociceptive effects of systemically-injected mibefradil are involved in the T-type calcium channels in peripheral nociceptors[6]. However, those data are established on the basis that mibefradil is a low selective inhibitor of T-type calcium. Actually, mibefradil has no selectivity to the subtype of T–type calcium channels, and can inhibit other calcium channels such as N- or L-type calcium when its dose is sufficient.
In this study, T-subtype calcium channel mRNA expression was inhibited by intrathecal administration of antisense oligonucleotide. These results demonstrate that the inhibition of Cav3.2 and Cav3.3 mRNA expression reverses the tactile allodynia and thermal hyperalgesia of the rats induced by CCD operation. In accordance with the behavior data, intrathecal administration of Cav3.2 and Cav3.3 antisense oligonucleotide decreases their protein expression respectively while we have not detected the Cav3.1 protein. The reason why different subtype T-type calcium channels have different roles may be involved in their distribution. Whereas the nervous system expresses many subtypes of T-type calcium channels, they are absent from Cav3.1 T channels in the spinal cord and DRG[16]. Indeed, the Cav3.1 subtype has an antinociceptive role. Recent findings[17] in knockout mice that lack Cav3.1 T-type calcium channels show that these animals exhibit an increased sensitivity to visceral pain, which indicates that the Cav3.1 T-type calcium channels support an antinociceptive mechanism. Electrophysiological recordings of ventroposterolateral thalamocortical neurons reveal a single spike pattern after the induction of visceral pain in mutant mice, whereas neurons from wild-type mice display bursts of activity. Hence, the activation of the Cav3.1 T-type calcium channels in the thalamus appears to inhibit visceral pain response, thus contributing to the perception of visceral hypersensitivity.
This study shows that the inhibition of Cav3.2 and Cav3.3 mRNA expression can reverse tactile allodynia and thermal hyperalgesia. Those results indicate that Cav3.2 and Cav3.3 have a pronociceptive role in neuropathic pain. The biophysical characteristics of the T-type channels have led to its implication mainly in the regulation of cell excitability. Since T-type channel activation occurs close to resting potential, they allow calcium influx when cells are at rest in response to subthreshold synaptic inputs. Thus, these channels enhance neuronal excitability and contribute to the generation of subthreshold membrane potential oscillations that lead to bursts of sodium dependent action potentials. Inhibition of the Cav3.2 and Cav3.3 calcium channels would result in an overall reduction in the underlying level of neuronal excitability, rendering the achievement of threshold levels of membrane depolarization less likely.
In conclusion, the present study shows that the Cav3.2 and Cav3.3 calcium channels in the spinal cord are involved in the development of allodynia and hyperalgesia in CCD rat model of neuropathic pain. Using something similar to the Cav3.2 and Cav3.3 blocker could be a good strategy to treat neuropathic pain.
References
- Woolf CJ, Salter MW. Neuronal plasticity: increasing the gain in pain. Science 2000;288:1765-9.
- Bridges D, Thompson SWN, Rice ASC. Mechanisms of neuropathic pain. Br J Anaesth 2001;87:12-26.
- Perez-Reyes E. Molecular physiology of low-voltage-activated t-type calcium channels. Physiol Rev 2003;83:117-61.
- Bertolino M, Llinas RR. The central role of voltage-activated and receptor-operated calcium channels in neuronal cells. Annu Rev Pharmacol Toxicol 1992;32:399-421.
- Kostyuk PG. Low-voltage activated calcium channels: achievements and problems. Neuroscience 1999;92:1157-63.
- Slobodan MT, Adam M, Vesna JT. Mechanical and thermal antino-ciception in rats following systemic administration of mibefradil, a T-type calcium channel blocker. Brain Res 2002;951:336-40.
- Ikeda, Heinke HB, Ruscheweyh R, Sandkuhler J. Synaptic plasticity in spinal lamina I projection neurons that mediate hyper-algesia. Science 2003;299:1237-40.
- Dogrul A, Gardell LR, Ossipov MH, Tulunay FC, Lai J, Porreca F. Reversal of experimental neuropathic pain by T-type calcium channel blockers. Pain 2003;105:159-68.
- Kim C, Jun K, Lee T, Kim SS, McEnery MW, Chin H, et al. Altered nociceptive response in mice deficient in the alpha (1B) subunit of the voltage-dependent calcium channel. Mol Cell Neurosci 2001;18:235-45.
- Yaksh TL, Rudy TA. Chronic catheterization of the spinal subarachnoid space. Physiol Behav 1976;17:1031-6.
- Hu SJ, Xing JL. An experimental model for chronic compression of dorsal root ganglion produced by intervertebral foramen stenosis in the rat. Pain 1998;77:15-23.
- Hargreaves K, Dubner R, Brown F, Flores C, Joris J. A new and sensitive method for measuring thermal nociception in cutaneous hyperalgesia. Pain 1988;32:77-88.
- Chaplan SR, Bach FW, Pogrel JW, Chung JM, Yaksh TL. Quantitative assessment of tactile allodynia in the rat paw. J Neurosci Methods 1994;53:55-63.
- Khosravani H, Altier C, Simms B, Hamming KS, Snutch TP, Mezeyova J. Gating effects of mutations in the Cav3.2 T type calcium channel associated with childhood absence epilepsy. J Biol Chem 2004;279:9681-4.
- Todorovic SM, Jevtovic-Todorovic V, Meyenburg A, Mennerick S, Perez-Reyes E, Romano C, et al. Redox modulation of T-type calcium channels in rat peripheral nociceptors. Neuron 2001;31:75-85.
- Beedle AM. Agonist-independent modulation of N-type calcium channels by ORL1 receptors. Nat Neurosci 2004;7:118-25.
- Kim D. Thalamic control of visceral nociception mediated by T-type Ca2+ channels. Science 2003;302:117-9.