Influence of CYP3A5 genetic polymorphism on cyclosporine A metabolism and elimination in Chinese renal transplant recipients
Introduction
Cyclosporine was the most frequently used immunosuppressant in organ transplant patients. However, it has a narrow therapeutic index which demands therapeutic drug monitoring followed by dose adjustment. Furthermore, the pharmacokinetic characteristics of cyclosporine were subject to high inter-patient variations.
The interindividual differences in the expression of drug metabolizing enzymes were the major factor contributing to pharmacokinetic variations[1]. The cytochrome P450 3A (CYP3A) subfamilies are the most abundant and important drug-metabolizing enzymes in humans and participate in the metabolism of 45%–60% of currently used drugs and a variety of other compounds[2]. A wide interindividual variability in CYP3A expression and function has been identified, and polymorphic expression of CYP3A5 was one of the major contributors[3,4]. Single nucleotide polymorphisms (SNP) in CYP3A5*3 and CYP3A5*6 that cause alternative splicing and protein truncation resulted in the absence of CYP3A5 from the tissues of some individuals[4,5]. CYP3A5 was believed to be the most important genetic contributor to interindividual and interracial differences in CYP3A-dependent drug clearance and responsive to many medicines[5]. Since immunosuppressant cyclosporine A (CsA) was a known substrate for CYP3A5[6], we sought to investigate whether the CYP3A5*3 polymorphism, which accounts for more than 70% of gene frequency in Chinese people, is associated with CsA metabolism and elimination in Chinese renal transplant patients.
Although the influence of CYP3A5 polymorphism on the pharmacokinetics of cyclosporine had been studied and reported by several research groups, the results and conclusions have been controversial[7–10]. Furthermore, most of the reported studies determined only the dose-adjusted trough concentrations, and no one had an insight into metabolite formation. In fact, it has been recognized that the CsA concentration, or dose requirement, was poorly associated with the clinical outcomes[11]. Therefore, we determined both CsA and its metabolite concentrations for evaluating the influence of the CYP3A5 polymorphism on cyclospo-rine’s elimination and metabolism. The metabolic ratio (MR) of CsA vs metabolites was used as an indicator showing the integrated effect of the CYP3A5 polymorphism on CsA metabolism and elimination.
Materials and methods
Human subjects The study protocol was approved by the ethical committee of the General Hospital of Nanjing Military Area. One-hundred-and-forty-seven Chinese (Han nationality) renal transplant recipients receiving CsA were genotyped for CYP3A5*3 polymorphism. All subjects (at least 6 months after first transplants) received the same immunosuppressive treatment (cyclosporine and dexamethasone), and took no drugs known to interfere with CsA disposition. The selected subjects had stable kidney graft function and laboratory values, without significant or new abnormal findings in physical examination or medical history. The CsA dose (Sandimmun Neoral, Novartis, Beijing, China; microemulsion formulation) was required to be constant during the last 5 d before the onset of the study. Blood samples from all the patients prior to the next dose were collected for determining CsA trough concentrations. The patients (38 subjects) who had good clinical compliance and whose age ranged from 30 to 40 were selected for the total metabolite determination. The demographic data of the subjects are summarized in Table 1.
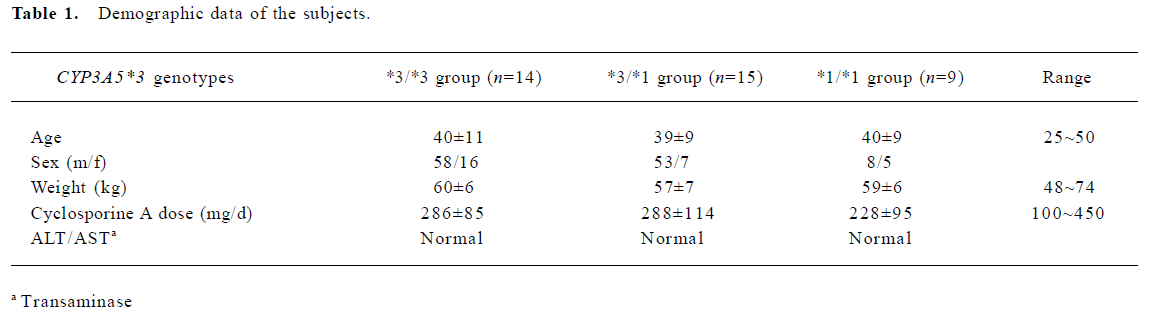
Full table
DNA isolation and genotyping The whole blood samples were obtained by venous puncture from the subjects and DNA was extracted from each sample (250 µL) using a DNA isolation kit (provided by Anhui-Gene Biotechnology Co Ltd, Hefei, China). The CYP3A5*3 genotype was then determined by polymerase chain reaction and amplification of specific alleles (PCR-ASA)[7]. The primers of CYP3A5*3 were designed according to its sequence in GENBANK (AC005020); the PCR-ASA conditions used in the genotyping are listed in Table 2 and Table 3, respectively. PCR-ASA was performed using a Perkin-Elemer GeneAmp PCR System 2400 (Wellesley, MA, USA). Representative detection of the CYP3A5*3 polymorphism by PCR-ASA is depicted in Figure 1.
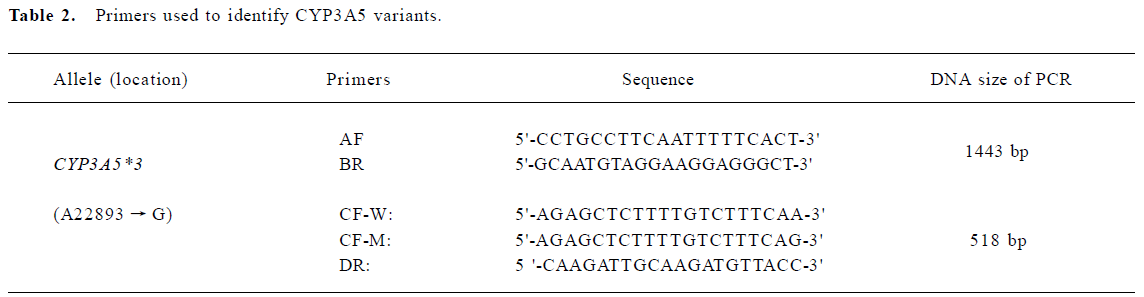
Full table
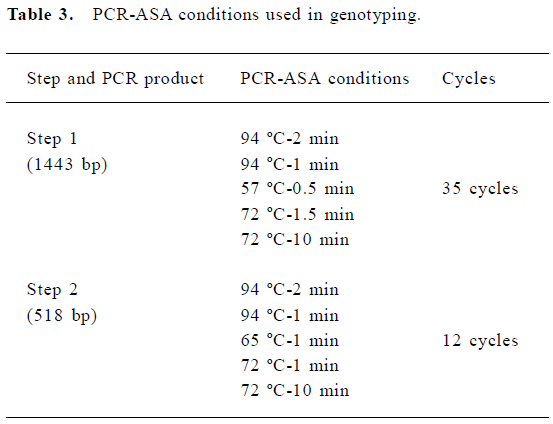
Full table
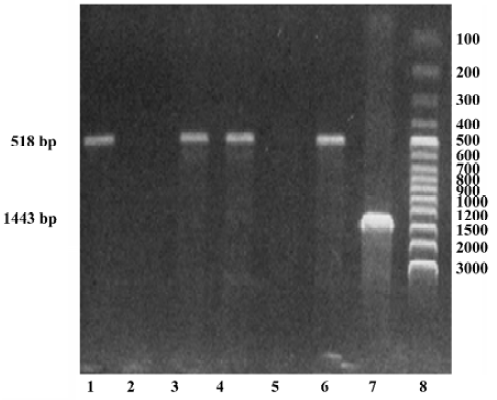
Determination of CsA and metabolite concentrations The whole blood concentrations of CsA and its total metabolites were measured by fluorescence polarization immunoassay[12] run on a TDX analyzer (Abbott, Abbott Park, Illinois, USA) following the manufacturer’s instructions. The whole blood reagent packs for CsA and metabolites were purchased from Abbott. The assay intraday and interday variations were determined to be within 15% and accuracy was close to 100%.
The 38 selected subjects were divided into the CYP3A5*3/*3 group (n=14), CYP3A5*1/*3 group (n=15) and CYP3A5*1/*1 group (n=9) according to genotyping. Both concentrations of cyclosporine and its metabolites were dose adjusted. The MR value was calculated by using the following equation[8]:
MR=CCsA (mg·L-1)/CMet (mg·L-1)
Statistical analysis In order to differentiate the potential influence of CYP3A5 genotype on the metabolism and the elimination of cyclosporine, we separately compared the dose-adjusted trough concentrations of the parent drug and its metabolites and the MR. Samples were grouped according to their CYP3A5*3 genotypes, and the statistical differences were determined using one-way ANOVA followed by post hoc multiple comparisons based on the least squares means (SAS 6.12).
Results
Genotyping Of the 147 patients in the study, 74 were found to be CYP3A5*3 homozygous carriers, 60 were CYP3A5*1*3 carriers and only 13 patients were the wild-type *1*1 carriers. The CYP3A5*3 gene frequency was determined to be 71% in this study in accordance with previously published reports. From the selected 38 subjects for further metabolite determination, 14, 15 and 9 were determined to be CYP3A5*3/*3, CYP3A5*1/*3 and CYP3A5*1/*1, respectively.
Trough concentrations of CsA, metabolites and MR The trough concentration adjusted with the dose was significantly higher in the wild allele carriers as compared to both the homozygous (*3*3) and heterozygous variants (*1*3). However, no significant difference was found when the dose-adjusted metabolite concentrations were compared between the different groups. Based on the trough concentrations of CsA and its metabolites, the MR values were calculated. The MR values for the 3 genotype groups were as follows: 0.92±0.62 for CYP3A5*3/*3 (n=14), 0.99±0.51 for CYP3A5*1/*3 (n=15), and 1.45±0.62 for CYP3A5*1/*1 (n=9), respectively. The result of the ANOVA analysis indicated weak statistical evidence of difference among the 3 groups (P=0.0882). Further post hoc comparisons with least squares means showed that only the MR values between the CYP3A5*3/*3 group and the CYP3A5*1/*1 group were significantly different (P=0.0328; Table 4, 5).
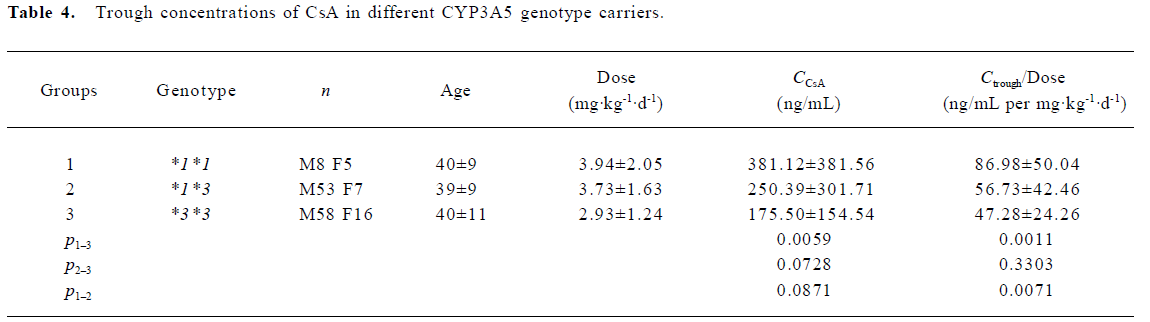
Full table
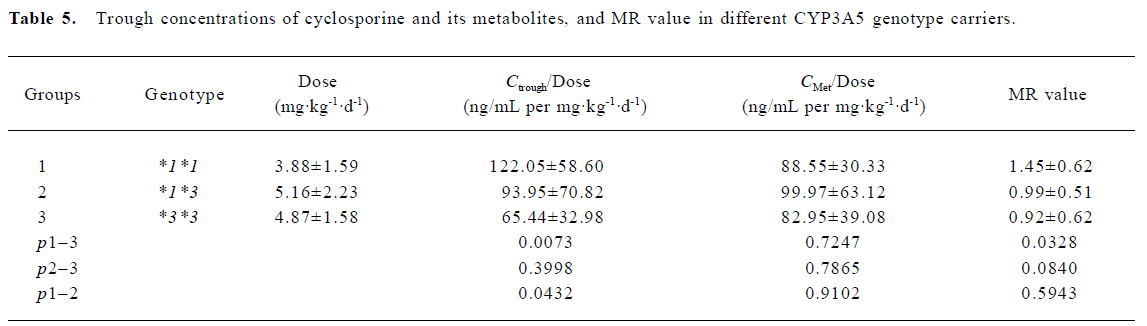
Full table
Discussion
The CYP3A5*3 variants were known to cause a significant lower hepatic and jejunal CYP3A5 mRNA expression as compared to the CYP3A5*1*1 genotype[13]. However, research on the influence of CYP3A5 gene polymorphism on CYP3A enzyme activity have been greatly inconsistent[7–10]. Some studies conducted in vivo supported that the CYP3A5*3 genotype resulted in slower CsA oral clearance as compared to the wild-type allele. In contrast, Yates et al reported that CsA oral clearance was significantly 37.87% lower in the CYP3A5*1*1 genotype compared to the CYP3A5*3*3 genotype (30.1±2.8 vs 41.5±3.1L/h, P=0.027)[14]. The possible cause for such a contradictory conclusion may be that the different parameters such as dose-adjusted trough concentrations, dose adjusted area under the curve (AUC) and dose requirements were compared in the different research. The contradictory results obtained from different parameters highlighted the importance of selecting more accurate parameters for accessing the influence of genotype on pharmacokinetics.
In the present study, we determined both CsA and its metabolite concentrations. The statistical comparison of the dose-adjusted metabolite concentrations showed no significant difference among the 3 CYP3A5 genotypes. However, significant differences were found when trough concentrations of CsA were compared among the 3 groups or between the wild-type and CYP3A5*3 variant carriers. These results indicate that the CYP3A5*3 variants exert no significant influence on the metabolism of cyclosporine. However, it seems to be controversial that the trough concentrations of CsA were significant lower in the CYP3A5*3 carriers compared to the wild-type CYP3A5 carriers. The possible influence of gender on the CsA pharmacokinetics and metabolism were statistically compared within the 3 CYP3A5 genotype groups and no significant difference was observed, which was inconsistent with the previous report[15].
It was argued that lower plasma concentrations of parent drugs might be caused by a more rapid metabolism (including first pass metabolism which occurs in the intestines or liver) or elimination itself. Cyclosporine was a well known substrate of P-glycoprotein of which the higher expression was the main cause for the lower bioavailability or faster oral clearance of cyclosporine. MDR1 (multidrug resistance gene), the gene encoding P-glycoprotein was also subject to high frequency of polymorphism. It was reported that CsA oral clearance was significantly higher in subjects who carried the 3435T allele as compared to the wild-type individuals (40.0±2.2 vs 26.4±3.1 L/h, P= 0.007), and the MDR1 C3435T genotype accounted for approximately 43% of the interindividual variability in CsA oral disposition. It was interesting to find that the CYP3A5*3 allele carriers were more likely to carry the MDR1 3435T allele than the 3435C allele (P=0.038); CYP3A5*3 G/G genotype individuals were 3435T allele carriers[14]. Combining the previous reports and our present study, we can conclude that the CYP3A5 polymorphism exerts little effect on cyclosporine metabolism; however, mechanisms underlying the CYP3A5*3-associated rapid clearance remains unknown.
Furthermore, interindividual differences in the systemic profile of cyclosporine metabolites were found to be responsible to the divergent adverse drug response to a therapeutic systemic CsA exposure level[16]. Therefore, determining only the trough concentrations or the AUC of CsA was not enough for therapeutic monitoring. We highlighted in the present study that MR values, taking both the parent drugs and metabolites into account, should be a more accurate indicator for therapeutic monitoring and for the evaluation of gene polymorphism influence on drug exposure. The statistical data of our study indicates that only the MR values between CYP3A5*3*3 and CYP3A5*1*1 group are significantly different (P=0.0328), with MR values in the *3*3 carriers 36.03% lower than that of *1*1 carriers.
In conclusion, the CYP3A5*3 polymorphism exerted little effect on cyclosporine metabolism. The MR should be a more accurate parameter for determining the body exposure of both parent drugs and potential active metabolites.
References
- Guengerich FP. Reactions and significance of cytochrome P-450 enzymes. J Biol Chem 1991;266:10019-22.
- Evans WE, Mcleod HL. Pharmacogenomics–drug disposition, drug targets, and side effects. N Engl J Med 2003;348:538-49.
- Shih PS, Huang JD. Pharmacokinetics of midazolam and 1'-hydroxymidazolam in Chinese with different CYP3A5 genotypes. Drug Metab Dispos 2002;30:1491-6.
- Hustert E, Haber M, Burk O, Wolbold R, He YQ, Klein K, et al. The genetic determinants of the CYP3A5 polymorphism. Pharmacogenetics 2001;11:773-9.
- Kuehl P, Zhang J, Lin Y, Lamba J, Assem M, Schuetz J, et al. Sequence diversity in CYP3A promoters and characterization if the genetic basis of polymorphic CYP3A5 expression. Nat Genet 2001;27:383-91.
- Min DI, Ku YM, Vichiendilokkul A, Fleckenstein LL. A urine metabolic ratio of dextromethorphan and 3-methoxymorphinan as a probe for CYP3A activity and prediction of cyclosporine clearance in healthy volunteers. Pharmacotherapy 1999;19:753-9.
- Hesselink DA, van-Schaik RH, van-der-Heiden IP, van-der-Werf M, Gregoor PJ, Lindemans J, et al. Genetic polymorphisms of the CYP3A4, CYP3A5, and MDR-1 genes and pharmacokinetics of the calcineurin inhibitors cyclosporine and tacrolimus. Clin Pharmacol Ther 2003;74:245-54.
- Anglicheau D, Thervet E, Etienne I, Hurault DLB, Le MY, Touchard G, et al. CYP3A5 and MDR1 genetic polymorphisms and cyclosporine pharmacokinetics after renal transplantation. Clin pharmacol Ther 2004;75:422-33.
- Hesselink DA, van-Gelder T, van-Schaik RH, Balk AH, van-der-Heiden IP, van Dam T, et al. Population pharmacokinetics of cyclosporine in kidney and heart transplant recipients and the influence of ethnicity and genetic polymorphism in the MDR-1, CYP3A4, and CYP3A5 genes. Clin Pharmacol Ther 2004;76:545-56.
- Haufroid V, Mourad M, Van KV, Wawrzyniak J, De Meyer M, Eddour DC, et al. The effect of CYP3A5 and MDR1 (ABCB1) polymorphism on cyclosporine and tacrolimus dose requirements and trough blood levels in stable renal transplant patients. Pharmacogenetics 2004;14:147-54.
- Fisher NC, Nightingale PG, Gunson BK, Lipkin GW, Neuberger JM. Chronic renal failure following liver transplantation: a retrospective analysis. Transplantation 1998;66:59-66.
- Wallemacq PE, Alexandre K. Evaluation of the new AxSYM cyclosporine assay: comparison with TDx monoclonal whole blood and emit cyclosporine assays. Clin Chem 1999;45:432-35.
- Williams JA, Cook J, Susan I, Hurst SI. A significant drug-metabolizing role for CYP3A5? Drug Metab Dispos 2003;31:1526-30.
- Yates CR, Zhang W, Song P, Li S, Gaber AO, Kotb M, et al. The effect of CYP3A5 and MDR1 polymorphic expression on cyclosporine oral disposition in renal transplant patients. J Clin Pharmacol 2003;43:555-64.
- Aros CA, Ardiles LG, Schneider HO, Flores CA, Alruiz PA, Jerez VR, et al. No gender-associated differences of cyclosporine pharmacokinetics in stable renal transplant patients treated with diltiazem. Transplant Proc 2005;37:3364-6.
- Christians U, Sewing KF. Alternative cyclosporine metabolic pathways and toxicity. Clin Biochem 1995;28:547-59.