Stimulation of melanogenesis by scoparone in B16 melanoma cells1
Introduction
The natural tanning process occurs as a response to exposure to UV radiation. This process is characterized by an increased number of melanocytes in the basal layer of the epidermis, increased size and number of melanosomes, increased production of melanin in melanosomes, increased dendricity of melanocytes, increased transport of melanosomes from melanocytes to keratinocytes, increased proliferation of keratinocytes and the thickening of the epidermis and stratum corneum. Keratinocytes with melanosomes provide protection from subsequent UV radiation exposure by scattering incoming light and absorbing UV radiation-generated free radicals in cells[1]. However, UV-induced tanning can cause damage of DNA and other cellular molecules, leading to mutagenesis, carcinogenesis, altered immunological responses and photoageing. A highly photoprotective tan that does not require skin damage and does not require altered metabolism might be highly beneficial.
Melanin synthesis is regulated by melanogenic enzymes such as tyrosinase, tyrosinase-related protein 1 (TRP-1), and tyrosinase-related protein 2 (TRP-2)[2]. Tyrosinase is a bifunctional enzyme that plays a pivotal role in the modulation of melanin production, first by catalyzing the hydroxylation of tyrosine to 3,4-dihydroxyphenylalanine (DOPA) and second, by catalyzing the oxidation of DOPA to DOPA-quinone[3]. TRP-2, which functions as DOPAchrome tautomerase, catalyzes the rearrangement of DOPAchrome to 5,6-dihydroxyindole-2-carboxylic acid (DHICA)[4], and TRP-1 oxidizes DHICA to a carboxylated indole-quinone[5]. Both work at downstream points of tyrosinase in the melanin biosynthetic pathway.
During the screening process, while looking for compounds that influence melanogenesis, we observed that scoparone (6,7-dimethoxycoumarin), a derivative of coumarin, was a potent stimulator of melanogenesis. Coumarins comprise of a group of phenolic compounds widely distributed in natural plants[6]. They have recently attracted much attention because of their broad pharmacological activities. Scoparone has been shown to have biological properties that include the inhibition of xanthine oxidase, immunosuppressive effects, vasodilation, lipid lowering activity and antioxidant effects[7–12]. However, the direct effect of scoparone alone on the expression of regulatory enzymes involved in melanogenesis has not been reported yet. In the present study, we examined the direct effects of scoparone on proliferation and melanogenesis in cultured B16 melanoma cells.
Materials and methods
Cell culture B16 murine melanoma cells (kindly supplied by Professor DG KIM, Department of Internal Medicine, Chonbuk National University Medical School, Jeonju, Korea) were cultured in DMEM containing 10% fetal bovine serum, 100 U/mL penicillin, 0.1 mg/mL streptomycin and 0.25 µg/mL amphotericin B at 37 ºC in a humidified 95% air/5% CO2 atmosphere. Drug treatment began 24 h after seeding. Scoparone and 7-methoxycoumarin were obtained from Aldrich Chemical Co (Milwaukee, WI, USA), and other coumarin derivatives were obtained from Sigma Chemical Co (St. Louis, MO, USA). The cells were harvested 3 d later and the melanin content and tyrosinase activity were determined in triplicate for each treatment[13].
Melanin content measurement The melanin content of the cultured B16 cells was measured as described previous-ly[14]. The cells were washed twice with phosphate-buffered saline (PBS) and lysed with 20 mmol/L Tris-0.1% Triton X-100 (pH 7.5). Cell lysates were precipitated with the same amount of 20% trichloroacetic acid (TCA). After washing twice with 10% TCA, the pellets were treated with ethyl alcohol:diethyl ether (3:1) and diethyl ether successively. Samples were air-dried, dissolved in 1 mL of 0.85 mol/L KOH, and boiled for 15 min. After cooling, absorbance was measured with a spectrophotometer at 400 nm. The amount of cellular melanin was corrected according to the protein content of the samples. The protein concentration was determined by the method used by Bradford[15].
Assay of tyrosinase activity Tyrosinase activity was assayed as DOPA oxidase activity using a modified method described by Lerch[16]. The cells were washed twice with PBS and lysed with 20 mmol/L Tris-0.1% Triton X-100 (pH 7.5). Tyrosinase activity was analyzed spectrophotometrically by following the oxidation of DOPA to DOPAchrome at 475 nm. The reaction mixture containing 100 µL of freshly prepared substrate solution [0.1% L-DOPA in 0.1 mol/L sodium phosphate (pH 6.0)] and 50 µL of enzyme solution was incubated at 37 ºC. The absorbance change was measured during the first 10 min of the reaction while the increase of the absorbance was linear, and corrections for auto-oxidation of L-DOPA in the controls were made. The specific activity of tyrosinase in the control cells was 0.37±0.01 mmol L-DOPA oxidized·min-1·mg-1 protein; activities were expressed as a percentage of the control cells.
MTT assay General viability of cultured cells was determined by the reduction of 3-(4,5-dimethylthiazol-2-yl)-2.5-diphenyltetrazolium bromide (MTT) to formazan[17]. After treatment, the cells were washed twice with PBS. MTT (100 µg/0.1 mL PBS) was added to each well. The cells were incubated at 37 ºC for 1 h and dimethyl sulfoxide (100 µL) was added to dissolve the formazan crystals. The absorbance was then measured at 570 nm with a spectrophotometer (Spectra MAX PLUS, Molecular Devices, Sunnyvale, CA, USA).
Western blotting The cells were homogenized in 100 µL of ice-cold lysis buffer (20 mmol/L Hepes; pH 7.2, 1% Triton X-100, 10% glycerol, 1 mmol/L phenylmethylsulfonyl fluoride, 10 µg /mL leupeptin, 10 µg /mL aprotinin). The homogenates containing 20 µg of protein were separated by SDS-PAGE with 10% resolving and 3% acrylamide stacking gel[18] and transferred to nitrocellulose sheets (Whatman, Brentford, Middlesex, UK) in a Western blot apparatus (Bio-Rad, Hercules, CA, USA) run at 50 V for 2 h. The nitrocellulose paper was blocked with 2% bovine serum albumin and then incubated for 4 h with 1 µg/mL goat IgG anti-murine tyrosinase (Santa-Cruz Biotechnology Inc, Santa-Cruz, CA, USA) or rabbit IgG anti-actin (Sigma, USA). The binding of antibodies was detected with anti-goat or anti-rabbit lgG conjugated with alkaline phosphatase (Sigma, USA). lmmunoblots were developed using a 5-bromo,4-chloro,3-indolylphos-phate/nitrobule tetrazolium (BCIP/NBT) solution (Pierce, Rockford, IL, USA).
Reverse transcription-polymerase chain reaction (RT-PCR) Total cellular ribonucleic acid (RNA) was prepared using Trizol solution (Gibco-BRL, Paisley, Scotland, UK) according to the manufacturer’s instructions. After the preparation of cDNA with oligo d(T) as a reverse transcriptase primer from the extracted RNA, amplification with PCR was performed using a GeneAmp kit (Perkin Elmer, Foster City, CA, USA). The oligonucleotide primers used for PCR were as follows: tyrosinase upstream 5'-GGC CAG CTT TCA GGC AGA GGT-3'; downstream 5'-TGG TGC TTC ATG GGC AAA ATC-3': TRP-1 upstream 5'-GCT GCA GGA GCC TTC TTT CTC-3'; downstream 5'-AAG ACG CTG CAC TGC TGG TCT-3': TRP-2 upstream 5'-GGA TGA CCG TGA GCA ATG GCC-3'; downstream 5'-CGG TTG TGA CCA ATG GGT GCC-3': actin upstream 5'-ACC GTG AAA AGA TGA CCC AG-3'; downstream 5'-TAC GGA TGT CAA CGT CAC AC-3'. cDNA amplification used the product of about 1 µg of the total RNA. The reaction was cycled 28 times for 60 s at 94 ºC, 60 s at 56 ºC and 60 s at 72 ºC. Fifty percent of the reaction mixture was analyzed by electrophoresis on 1% agarose gels and stained by ethidium bromide. In order to check the reproducibility of the results, each experiment was carried out 3 times.
Statistical analysis Values were expressed as Mean± SEM. All data were examined for their statistical significance with the Student’s t-test, one-way ANOVA and repeated measured ANOVA. Differences with P<0.05 were considered statistically significant.
Results
Methoxy group of the coumarin ring determined the melanogenesis To investigate the effect of coumarin derivatives on melanogenesis, B16 cells were incubated with 50 µg/mL of each compound for 72 h. Coumarin, 6,7-dihydroxycoumarin, 7-hydroxycoumarin showed little effect on melanogenesis, but the methoxylated coumarins, including 7-hydroxy-6-methoxycoumarin, 7-methoxycoumarin, and 6,7-dimethoxycoumarin, increased melanin content 3.1-, 5.8-, and 8.4-fold, respectively. These findings suggest that the 6- or 7-methoxy group is essential for enhancing melanogenesis (Figure 1). Since scoparone (6,7-dimethoxycoumarin) was found to be the most potent compound for stimulating melanogenesis, all additional experiments focused on this analog.
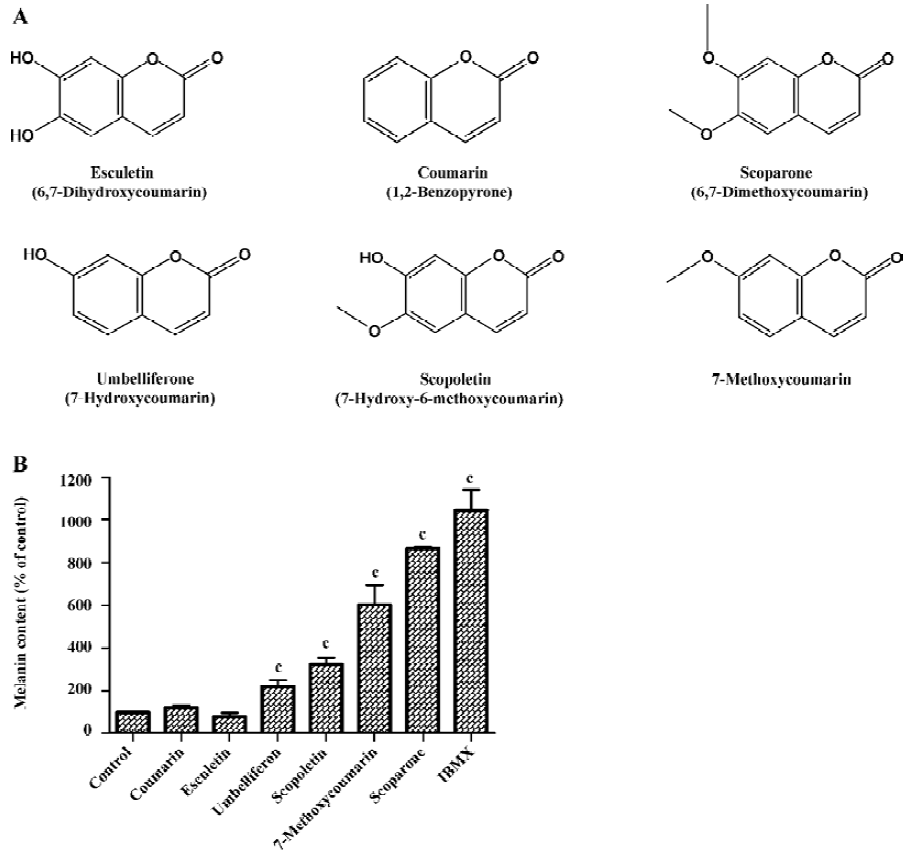
Scoparone increased the melanin content and tyrosinase activity in a dose-dependent manner After the exposure of the cultured B16 cells to scoparone or isobutylmethyl-xanthine (IBMX), their suspended cell colors were grossly darkened. Treatment with scoparone (50 µg/mL) resulted in a deep brown pigmentation, which correlated with increased melanin production (Figure 2A, lane 2). Its pigmentation was similar to the effect of IBMX, an inhibitor of phosphodiesterase, used as a positive control for the stimulation of melanogenesis (Figure 2A, lane 3). To determine the effect of scoparone on melanogenesis, B16 cells were exposed for 72 h to 3 concentrations of scoparone (12.5, 25 and 50 µg/mL). The cellular content of melanin and tyrosinase activity were increased by scoparone in a dose-dependent manner (Figure 2B). At a concentration of 50 µg/mL, the cellular melanin content and tyrosinase activity reached a near maximum level that was about an 8.5-fold increase for melanin and about 6.34-fold increase for tyrosinase activity compared to the control cells. The viability itself as assayed by MTT was unaffected by the presence of scoparone at this concentration.
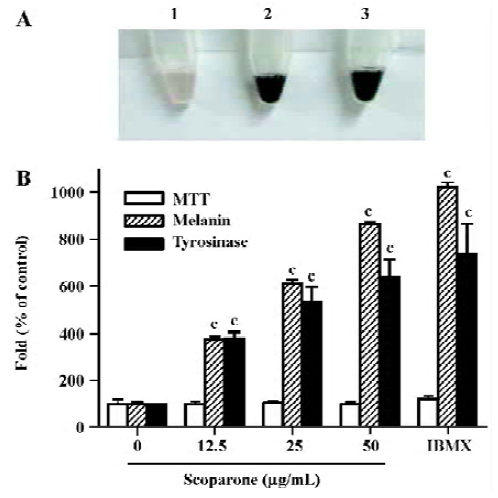
Scoparone increased melanin content and tyrosinase protein through cAMP signaling To demonstrate the involvement of cAMP signaling in scoparone-induced melanogenesis, a melanin content assay and Western blot for tyrosinase were performed in the presence of H-89, a cAMP-dependent protein kinase A (PKA) inhibitor[19]. As shown in Figure 3A, increased melanin content by scoparone and IBMX were completely abolished by the presence of 10 µmol/L H-89. Little cytotoxicity was observed at this concentration of H-89 as determined by MTT assay (data not shown).
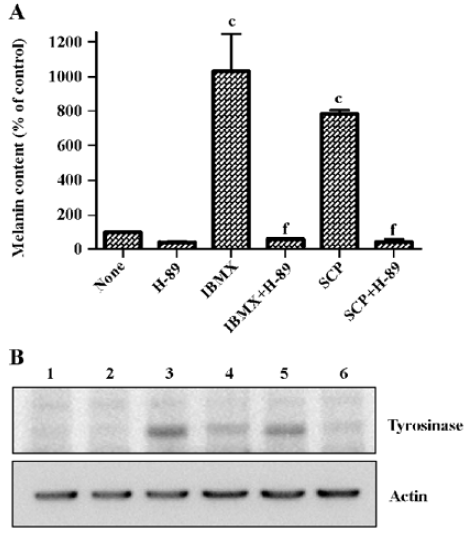
Increases of melanin content and tyrosinase activity by scoparone were accompanied by the increase of the tyrosinase protein (Figure 3B, lane 5). IBMX was used to induce tyrosinase expression as a positive control (Figure 3B, lane 3). H-89 also abolished the scoparone- or IBMX-induced increase of tyrosinase protein expression (Figure 3B, lanes 4 and 6), indicating the involvement of cAMP signaling in scoparone-induced melanogenesis.
Scoparone-regulated melanogenesis at the transcriptional level We also examined the effects of scoparone treatment on mRNA levels. The cells were treated with 50 µg/mL of scoparone for 72 h and the total cellular RNA was extracted. Specific mRNA was amplified after reverse transcription with PCR using specific primers for tyrosinase, TRP-1 and TRP-2. As shown in Figure 4, mRNA of tyrosinase, TRP-1 and TRP-2 increased after treatment with scoparone. These results suggest that scoparone-induced stimulation of melanogenesis occurs at the transcriptional level.
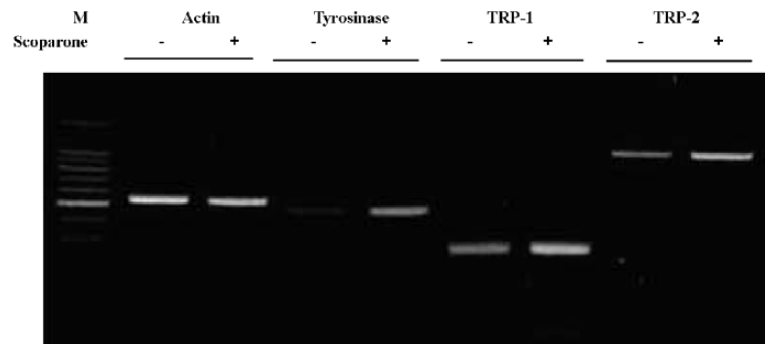
Discussion
Studies have demonstrated that extracts from some herbs such as glycyrrhizin[14] or quercetin[20], increase melano-genesis. In this study, we tried to further identify compounds affecting melanogenesis and demonstrated that scoparone stimulated melanogenesis in B16 cells in a dose-dependent manner. Among the many coumarin derivatives tested, 7-hydroxy-6-methoxycoumarin, 7-methoxycoumarin, and 6,7-dimethoxycoumarin (scoparone) treatment increased the melanin content in the cells studied. Other coumarins, including coumarin 6,7-dihydroxycoumarin, 7-hydroxy-coumarin, which is structurally similar to scoparone, had little effect on melanin production in the B16 cells. This suggests that the methoxy group, at the C-7 position (and possibly the C-6 position) in the coumarin ring, is essential for melanogenic activity. Actually, coumarin derivatives with hydroxyl group at the C-6 or C-7 positions have been reported to have inhibitory effects on tyrosinase activity[21].
Melanin is a unique pigmented biopolymer synthesized by specialized cells known as melanocytes, the dendritic cells in the skin, hair, eyes and other locations. Melanin has a number of important functions including determination of phenotypic appearance, protective coloration, balance and auditory processing, absorption of toxic drugs and chemicals, as well as neurological development during embryogenesis[1]. Melanogenesis itself is a complex process. In fact, the number of genes involved in regulating mammalian pigmentation is quite large, and at least 80 genetic loci have been identified for the regulation of melanogenesis either directly or indirectly[22]. Mutations of these genes have been shown to be associated with a number of different pigmentary diseases, including various forms of ocular and oculocuta-neous albinism, piebaldism, Hirschsprung’s disease and Waarden-berg’s syndrome[22].
Among these, the tyrosinase gene family has been shown to play an important role in the regulation of melanogenesis[23]. The tyrosinase gene family consists of tyrosinase TRP-1 and TRP-2. These molecules are glycoproteins embedded in the melanosome membrane that share a 70%–80% nucleotide sequence homology with 30%–40% amino acid identity; they also share common functional motifs such as epidermal growth factor receptor and copper binding sites[2]. As shown in the results, scoparone-induced melanogenesis was accompanied by the increase of activity, mRNA and protein expression of tyrosinase. In addition to tyrosinase, a key regulatory enzyme for melanogenesis, expressions of TRP-1 and TRP-2 were observed to be increased after treatment with scoparone, indicating the important roles of the tyrosinase gene family.
Recent investigation on the molecular mechanisms regulating pigmentation suggests that cAMP and protein kinase C (PKC) are the 2 major intracellular signaling molecules critical to pigmentation[24]. Extracellular signals increase cAMP levels, activating PKA. PKA then presumably alters the transcription rate of key genes including tyrosinase. Inactive PKC may also be activated by extracellular signals. Active PKC then phosphorylates tyrosinase, leading to its activation[25]. Recent reports suggest that the cGMP signal transduction pathway also stimulates melanogenesis[26,27].
cAMP-induced melanogenesis has been reported to be mediated by phosphorylation and the activation of the cAMP response element binding protein (CREB) family transcription factors by PKA. The binding of CREB to the promoter site of the microphthalamia-associated transcription factor (MITF) results in the indirect activation of tyrosinase promoter by MITF[25,28]. Increased melanin and tyrosinase protein expression by scoparone and IBMX were completely inhibited by the presence of H-89, an inhibitor of PKA. These results indicate that cAMP signaling pathways play a major role in scoparone-induced melanogenesis. Tumor necrosis factor-α inhibits both basal and cAMP-induced melanogenesis through the activation of nuclear factor κB (NF-κB)[29], and recently, scoparone was reported to inhibit NFκB activation[30]. While no NFκB activator was included in our experiments, further study is needed for the thorough understanding of the signal transduction pathway in scoparone-mediated stimulation of melanogenesis.
Currently many research efforts have focused on the specific mechanisms involved in melanogenesis in order to develop new therapeutic agents for skin pigmentation abnormalities. In this regard, the agents that stimulate tyrosinase activity and melanin production can also be used as skin tanning agents. Dihydroxyacetone (DHA)-containing preparations have been used for more than 50 years and are still very popular for temporary pigmentation resembling a UV-induced tan[31,32]. The tan produced by UVB and/or UVA radiation is photoprotective against subsequent UV exposure. DHA also produce cosmetically acceptable pigmentation of vitiliginous skin[33]. Application of the stimulatory effects of scoparone on melanogenesis might be useful as an adjunctive therapy for treatment of hypopigmentation-related disorders, as well as for tanning.
In summary, we have demonstrated that scoparone stimulates melanogenesis and tyrosinase activity. In addition, we have also found that scoparone-induced stimulation of melanogenesis occurs through increased expression of tyrosinase, TRP-1 and TRP-2 at the transcriptional level through PKA signaling.
References
- Hearing VJ. Regulation of melanin formation. In: Nordlund JJ, Boissy RE, Hearing VJ, King RA, Ortonne JP, editors. The pigmentary system: physiology and pathophysiology. New York: Oxford University Press; 1998. p423–38.
- del Marmol V, Beermann F. Tyrosinase and related proteins in mammalian pigmentation. FEBS Lett 1996;381:165-8.
- Hearing VJ, Jimenez M. Mammalian tyrosinase-the critical regulatory control point in melanocyte pigmentation. Int J Biochem 1987;19:1141-7.
- Yokoyama K, Yasumoto K, Suzuki H, Shibahara S. Cloning of the human DOPAchrome tautomerase/tyrosinase-related protein 2 gene and identification of two regulatory regions required for its pigment cell-specific expression. J Biol Chem 1994;269:27080-7.
- Kobayashi T, Urabe K, Winder A, Jimenez-Cervantes C, Imokawa G, Brewington T, et al. Tyrosinase related protein 1 (TRP1) functions as a DHICA oxidase in melanin biosynthesis. EMBO J 1994;13:5818-25.
- Egan D, O’kennedy R, Moran E, Cox D, Prosser E, Thornes RD. The pharmacology, metabolism, analysis and applications of coumarin and coumarin-related compounds. Drug Metabol 1990;22:503-29.
- Huang HC, Chu SH, Lee Chao PD. Vasorelaxants from Chinese herbs, emodin and scoparone, possess immunosuppressive pro-perties. Eur J Pharmacol 1991;198:211-3.
- Huang HC, Huang YL, Chang JL, Chen CC, Lee YT. Possible mechanism of immunosuppressive effect of scoparone (6,7-dimethoxycoumarin). Eur J Pharmacol 1992;217:143-8.
- Huang HC, Lee CR, Weng YI, Lee MC, Lee YT. Vasodilator effect of scoparone (6,7-dimethoxycoumarin) from a Chinese herb. Eur J Pharmacol 1992;218:123-8.
- Huang HC, Weng YI, Lee CR, Jan TR, Chen YL, Lee YT. Protection by scoparone against the alterations of plasma lipopro-teins, vascular morphology and vascular reactivity in hyperli-pidaemic diabetic rabbit. Br J Pharmacol 1993;110:1508-14.
- Mennes WC, Luijckx NB, Wortelboer HM, Noordhoek J, Blaauboer BJ. Differences in the effects of model inducers of cytochrome P450 on the biotransformation of scoparone in rat and hamster liver. Arch Toxicol 1993;67:92-7.
- Hoult JR, Paya M. Pharmacological and biochemical actions of simple coumarins: natural products with therapeutic potential. Gen Pharmacol 1996;27:713-22.
- Ando H, Ryu A, Hashimoto A, Oka M, Ichihashi M. Linoleic acid and alpha-linolenic acid lightens ultraviolet-induced hyperpigmentation of the skin. Arch Dematol Res 1998;290:375-81.
- Jung GD, Yang JY, Song ES, Park JW. Stimulation of melanogenesis by glycyrrhizin in B16 melanoma cells. Exp Mol Med 2001;33:131-5.
- Bradford MM. A rapid and sensitive method for the quantitation of microgram quantities of protein utilizing the principle of protein-dye binding. Anal Biochem 1976;72:248-54.
- Lerch K. Monophenol monooxygenase from Neurospora crassa. Methods Enzymol 1987;142:165-9.
- Mosmann T. Rapid colorimetric assay for cellular growth and survival: application to proliferation and cytotoxicity assays. J Immunol Methods 1983;65:55-63.
- Laemmli UK. Cleavage of structural proteins during the assembly of the head of bacteriophage T4. Nature 1970;227:680-5.
- Chijiwa T, Mishima A, Hagiwara M, Sano M, Hayashi K, Inoue T, et al. Inhibition of forskolin-induced neurite outgrowth and protein phosphorylation by a newly synthesized selective inhibitor of cyclic AMP-dependent protein kinase, N-[2-(p-bromo-cinnamylamino)ethyl]-5-isoquinolinesulfonamide (H-89), of PC12D pheochromocytoma cells. J Biol Chem 1990;265:5267-72.
- Nagata H, Takekoshi S, Takeyama R, Homma T, Yoshiyuki Osamura R. Quercetin enhances melanogenesis by increasing the activity and synthesis of tyrosinase in human melanoma cells and in normal human melanocytes. Pigment Cell Res 2004;17:66-73.
- Masamoto Y, Murata Y, Baba K, Shimoishi Y, Tada M, Takahata K. Inhibitory effects of esculetin on melanin biosynthesis. Biol Pharm Bull 2004;27:422-5.
- Hearing VJ. Biochemical control of melanogenesis and melano-somal organization. J Investig Dermatol Symp Proc 1999;4:24-8.
- Pawelek JM, Chakraborty AK. The enzymology of melano-genesis. In: Nordlund JJ, Boissy RE, Hearing VJ, King RA, Ortonne JP, editors. The pigmentary system: physiology and pathophy-siology. New York: Oxford University Press; 1998. p391–400.
- Busca R, Ballotti R. Cyclic AMP, a key messenger in the regulation of skin pigmentation. Pigment Cell Res 2000;13:60-9.
- Park HY, Gilchrest BA. Signaling pathways mediating melano-genesis. Cell Mol Biol 1999;45:919-30.
- Romero-Graillet C, Aberdam E, Biagoli N, Massabni W, Ortonne JP, Ballotti R. Ultraviolet B radiation acts through the nitric oxide and cGMP sigmal transduction pathway to stimulate melanogenesis in human melanocytes. J Biol Chem 1996;271:28052-6.
- Romero-Graillet C, Aberdam E, Clement M, Ortonne JP, Ballotti R. Nitric oxide produced by ultraviolet-irradiated keratinocytes stimulates melanogenesis. J Clin Invest 1997;99:635-42.
- Lee J, Jung E, Park J, Jung K, Park E, Kim J, et al. Glycyrrhizin induces melanogenesis by elevating a cAMP level in B16 melanoma cells. J Invest Dermatol 2005;124:405-11.
- Englaro W, Bahadoran P, Bertolotto C, Busca R, Derijard B, Livolsi A, et al. Tumor necrosis factor alpha-mediated inhibition of melanogenesis is dependent on nuclear factor kappa B activation. Oncogene 1999;18:1553-9.
- Jang SI, Kim YJ, Kim HJ, Lee JC, Kim HY, Kim YC, et al. Scoparone inhibits PMA-induced IL-8 and MCP-1 production through suppression of NF-kappaB activation in U937 cells. Life Sci 2006;78:2937-43.
- Levy S. Dihydroxyacetone-containing sunless or self-tanning lotions. J Am Acad Dermatol 1992;27:989-93.
- Levy S. Tanning preparations. Dermatol Clin 2000;18:591-6.
- Fesq H, Brockow K, Strom K, Mempel M, Ring J, Abeck D. Dihydroxyacetone in a new formulation –– a powerful therapeutic option in vitiligo. Dermatology 2001;203:241-3.