Synthesis and anti-diabetic activity of (RS)-2-ethoxy-3-{4-[2-(4-trifluoro-methanesulfonyloxy-phenyl)-ethoxy]-phenyl}-propionic acid
Introduction
Type 2 diabetes is a metabolic disease characterized by insulin resistance, hyperglycemia, and often hyperlipidemia. Untreated type 2 diabetes can lead to several chronic complications such as retinopathy, nephropathy, neuropathy, and cardiovascular diseases[1], the latter leading to increased mortality. Many peroxisome proliferator-activated receptor (PPARs) agonists, such as clofibrate, bezafibrate, pioglitazone and rosiglitazone, have been used as treatments for type 2 diabetes[2].
The PPAR are a group of 3 nuclear receptor isoforms[3,4], PPARα, PPARγ, and PPARβ(δ), encoded by different genes. They play critical physiological roles in the regulation of lipid and glucose metabolism. PPARα regulates lipid homeostasis via fatty acid catabolism, including fatty acid binding, uptake, and oxidation, as well as lipoprotein assembly and transport[5]. Fibrates (PPARα agonists; eg, fenofibrate and clofibrate) primarily decrease serum triglyceride levels and increase high density lipoprotein cholesterol (HDLc) levels, but they also improve glucose tolerance in type 2 diabetic patients[6] and reduce weight gain in rodents without affecting food intake[7]. PPARγ is an important component in the adipogenic signaling cascade and in lipid storage and utilization. Many synthetic compounds, such as the thiazolidinediones (TZD), have been discovered to function as high-affinity PPARγ agonists[8,9]. These selective PPARγ agonists are potent agents with the ability to improve insulin sensitivity and glucose tolerance, and normalize elevated plasma glucose and insulin levels in type 2 diabetic patients. But the improvement in insulin sensitivity is accompanied by body weight gain[10,11]. So, recently, there has been considerable interest in combining the beneficial activities of both PPARα and PPARγ activation, because the dual agonist approach should be well suited for the treatment of patients with type 2 diabetes[12–14]. Several dual PPARα/γ agonists have been discovered, such as AZ242, raglitazar, KRP-97 and GW-0072.
In the present study, we synthesized the compound (RS)-2-ethoxy-3-{4-[2-(4-trifluoromethanesulfonyloxy-phenyl)-ethoxy]-phenyl}-propionic acid (C20H21F3O7S), and determined that it was a dual PPARα/γ agonist and a potent antidiabetic agent.
Materials and methods
Chemical synthesis All melting points were determined using a Yanaco micromelting point apparatus without correction. 1H nuclear magnetic resonance (NMR) spectra were measured using a Varian Unity INOVA 300 MHz instrument. Mass spectrometry (MS) was carried out using an AutoSpec Ultima-TOF mass spectrometer. Column chromatography was performed on silica gel (Qingdao Haiyang Chemical Co, 200–300 mesh). A general strategy to synthesize compound I is shown in Scheme 1.
Preparation of 2-(4-benzyloxy-phenyl)-ethanol (com-pound 2) A solution of 2-(4-hydroxyphenyl)ethanol (1.38 g, 10 mmol), benzyl bromide (1.71 g, 10 mmol), and anhydrous potassium carbonate (1.66 g, 12 mmol) in dry acetone (50 mL) was heated under reflux for 8 h. After filtration the solution was concentrated, and water was added to the residue (10 mL). The neutral solution was extracted with AcOEt. The extract was washed with brine, dried over Na2SO4, and concentrated under vacuum. The residue was separated by silica gel column chromatography (hexanes/AcOEt=5:1) to give compound 2 (yield 1.63 g [78%]; Mp 78–79 °C; 1HNMR [CDCl3, 300 MHz] δ ppm: 7.445–7.320 [m, 5H, ArH], 7.15 [d, 2H, ArH, J=8.7 Hz], 6.93 [d, 2H, ArH, J=8.7 Hz], 5.051 [s, 2H, -CH2-], 3.828 [t, 2H, -CH2-, J=6.6Hz], 2.815 [t, 2H, -CH2-, J=6.6 Hz]).
Preparation of methanesulfonic acid 2-(4-benzyloxy-phenyl)-ethyl ester (compound 3) Compound 2 (2.28 g, 10 mmol) and triethyl amine (1.21 g, 12 mmol) were dissolved in methylene chloride and cooled to -10 °C. Methanesulfonyl chloride (1.38 g, 12 mmol) was then added at temperatures between -10 °C and -5 °C. The solution was stirred at room temperature for 6 h. The filtrate was washed with saturated NaHCO3 and water. The methylene chloride was evaporated and the residue was separated by silica gel column chromatography (hexanes/AcOEt=5:1) to give compound 3 (yield 2.45 g [80%]; Mp 66–68 °C; 1HNMR [CDCl3, 300 MHz] δ ppm: 7.437–7.322 [m, 5H, ArH], 7.15 [d, 2H, ArH, J=8.7 Hz], 6.93 [d, 2H, ArH, J=8.7 Hz], 5.053 [s, 2H, -CH2-], 4.377 [t, 2H, -CH2-, J=6.9 Hz], 2.992 [t, 2H, -CH2-, J=6.9 Hz], 2.828 [s, 3H, -CH3]).
Preparation of (RS)-3-{4-[2-(4-benzyloxy-phenyl)-ethoxy]-phenyl}-2-ethoxy-propionic acid ethyl ester (com-pound 4) To a solution of ethyl (RS)-2-ethoxy-3-(4-hydroxy-phenyl)-propanoate (1.19 g, 5 mmol) in acetonitrile (60 mL) were added potassium carbonate (2.07 g, 15 mmol) and magnesium sulfate (0.41 g, 3 mmol). The resulting mixture was added to the acetonitrile solution of compound 3 (2.53 g, 8.2 mmol) and refluxed for 8 h. The filtrate was extracted with AcOEt. The extract was washed with brine, dried, and concentrated. The residue was separated by silica gel column chromatography (hexanes/AcOEt=5:1) to give compound 4 (yield 1.15 g [51%]; 1HNMR [CDCl3, 300 MHz] δ ppm: 7.450–7.324 [m, 5H, ArH], 7.20 [d, 2H, ArH, J=8.1Hz], 7.14 [d, 2H, ArH, J=8.7 Hz], 6.93 [d, 2H, ArH, J=8.1 Hz], 6.81 [d, 2H, ArH, J=8.7 Hz], 5.056 [s, 2H, -CH2-], 4.202–4.085 [m, 4H, 2CH2], 3.964 [t, 1H, -CHCO-, J=6.6 Hz], 3.649–3.296 [m, 2H, -OCH2-], 3.051–3.005 [m, 2H, -CH2-], 2.95 [d, 2H, ArCH2-, J= 6.6 Hz], 1.251–1.142 [m, 6H, 2CH3]).
Preparation of (RS)-3-{4-[2-(4-hydroxy-phenyl)-ethoxy]-phenyl}-2-ethoxy-propionic acid ethyl ester (compound 5) A mixture of compound 4 (2 g, 4.5 mmol), 0.2 g of 10% Pd/C, and 50 mL of THF was hydrogenated in a Parr apparatus until uptake of H2 ceased. The mixture was filtered and the filtrate was concentrated to give compound 5 (yield 1.45 g [90%]; Mp 108–109 °C; 1HNMR [CDCl3, 300 MHz] δ ppm: 7.160–7.123 [m, 4H, ArH], 6.820–6.766 [m, 4H, ArH], 4.198–4.075 [m, 4H, 2CH2], 3.958 [t, 1H, -CHCO-, J=6.6 Hz], 3.643–3.292 [m, 2H, -OCH2-], 3.032–2.985 [m, 2H, -CH2-], 2.94 [d, 2H, ArCH2-, J=6.6 Hz], 1.247–1.136 [m, 6H, 2CH3]).
Preparation of (RS)-2-ethoxy-3-{4-[2-(4-trifluoro-methanesulfonyloxy-phenyl)-ethoxy]-phenyl} -propionic acid ethyl ester (compound 6) To a cooled solution of compound 5 (1.79 g, 5 mmol) and triethyl amine (1.01 g, 10 mmol) in CH2Cl2 (60 mL) was added trifluoromethanesulfonyl chloride (1.68 g, 10 mmol) at -10 °C and -5 °C. The solution was stirred at room temperature for 6 h, then the filtrate was washed with CH2Cl2. The organic layer was evaporated and the residue was separated by silica gel column chromatography (hexanes/AcOEt=6:1) to give compound 6 (yield 2.13 g [86.9%]; 1HNMR [CDCl3, 300 MHz] δ ppm: 7.36 [d, 2H, ArH, J=8.1 Hz], 7.20 [d, 2H, ArH, J=8.1 Hz], 7.15 [d, 2H, ArH, J=8.1 Hz], 6.80 [d, 2H, ArH, J=8.1 Hz], 4.200–4.128 [m, 4H, 2CH2], 3.969 [t, 1H, -CHCO-, J=6.6 Hz], 3.651–3.295 [m, 2H, -OCH2-], 3.121–3.078 [m, 2H, -CH2-], 2.95 [d, 2H, ArCH2-, J=6.6 Hz], 1.246–1.138 [m, 6H, 2CH3]).
Preparation of (RS)-2-ethoxy-3-{4-[2-(4-trifluoro-methanesulfonyloxy-phenyl)-ethoxy]-phenyl} -propionic acid (compound I) To a solution of compound 6 (490 mg, 1 mmol) in ethanol (20 mL) was added lithium hydroxide (23.95 mg, 1 mmol). The mixture was stirred for 3 h and evaporated. The residue was dissolved in water and acidified, then the solution was extracted with AcOEt. The extract was washed, dried, and concentrated. The residue was separated by silica gel column chromatography (hexanes/AcOEt=3:1) to give compound I (yield 323 mg [70%]; 1HNMR [CDCl3, 300 MHz] δ ppm: 7.36 [d, 2H, ArH, J=8.7 Hz], 7.21 [d, 2H, ArH, J=8.7 Hz], 7.16 [d, 2H, ArH, J=8.7 Hz], 6.81 [d, 2H, ArH, J=8.7 Hz], 4.153 [t, 2H, -CH2-, J=6.3 Hz], 4.053–4.012 [m, 1H, -CHCO-], 3.663– 3.367 [m, 2H, -OCH2-], 3.104 [t, 2H, -CH2-, J=6.3 Hz], 3.042–2.910 [m, 2H, ArCH2-], 1.166 [t, 3H, -CH3]; ESI [C20H21F3O7S] m/z: 461.1 [-Q], 414.9, 149.1.
In vitro transactivation assays cDNAs for Human RXR and PPAR were obtained by reverse transcription-polymerase chain reaction (RT-PCR) using human liver or adipose tissue[15]. Amplified cDNAs were cloned into the pcDNA3.1 expression vector and the inserts were confirmed by sequencing. U2OS cells were cultured in McCoy’s 5A media with 10% heat-inactivated fetal bovine serum (FBS) in a humidified 5% CO2 atmosphere at 37 °C. Cells were seeded in 96-well plates the day before transfection to give a confluence of 50%–80% at transfection. A total of 60 ng of DNA containing 10 ng of hRXR alpha, 10 ng of pCMV Gal, 10 ng of nuclear receptor expression vectors and 30 ng of the corresponding reporters were cotransfected per well using the FuGene6 transfection reagent according to the manufacturer’s instructions (Roche). Twenty-four hours after transfection, cells were incubated with 10% charcoal-stripped FBS DMEM and were treated with compound I dissolved in dimethylsulfoxide (DMSO). The final concentration of DMSO in culture medium was 0.1%. Cells were treated with compound I for 24 h, and then collected with cell culture lysis buffer. Luciferase activity was monitored using a luciferase assay kit (Promega) according to the manufacturer’s instructions. Light emission was read in a Labsystems Ascent Fluoroskan reader. To measure galactosidase activity to normalize the luciferase data, 50 μL of supernatant from each transfection lysate was transferred to a new microplate. Galactosidase assays were performed in microwell plates using a kit from Promega and read in a microplate reader. Concentration-effect curves are presented in Figure 1.
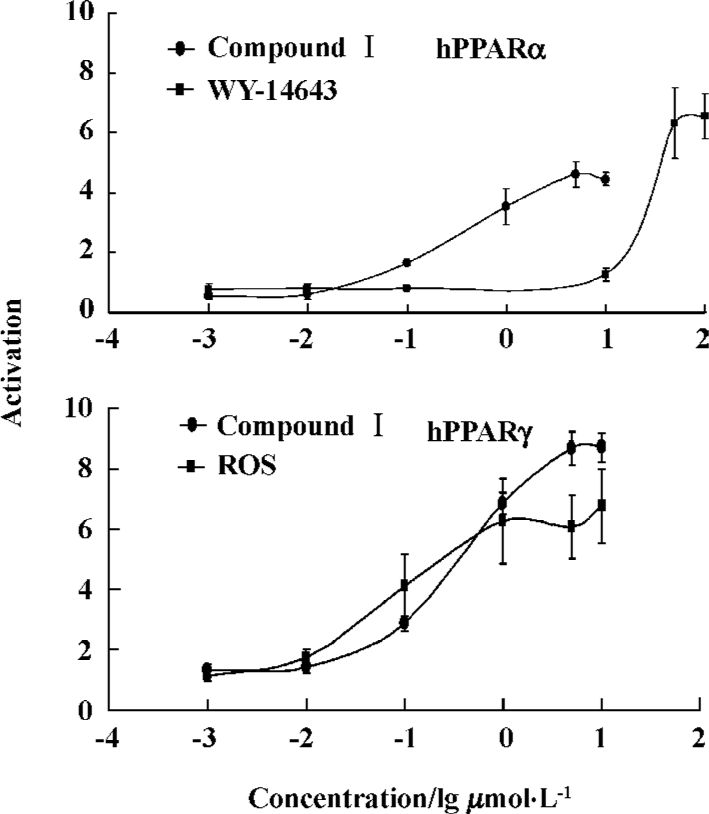
Animals and treatment Normal ICR mice and insulin-resistant KKAy mice were purchased from the Experimental Animal Center of the Chinese Academy of Medical Sciences, Beijing, China. KKAy mice are known to be excellent models of obese insulin-resistant type 2 diabetes, and ICR mice were used as the normal group. All mice were individually housed with a 12 h/12 h light-dark cycle and were provided with a high-fat diet and water ad libitum. At the beginning of the study, KKAy mice were weighed, bled via the tail vein in the fasted state (4 h), and sorted into 4 treatment groups (n=10 each) based on the decrease of blood glucose in the insulin tolerance testing, glucose levels, blood triglyceride levels and initial body weight. From the next day, KKAy mice were orally administered vehicle (water, 0.5% Tween-80), 7 μmol·kg-1·d-1 rosiglitazone, 7 μmol·kg-1·d-1 AZ242 or 7 μmol·kg-1·d-1 compound I. Normal ICR mice (n=10) were also treated with vehicle. Experiments were performed during treatment, and the present study was conducted in accordance with ethical guidelines (Institute of Materia Medica, Chinese Academy of Medical Sciences & Peking Union Medical College) for animal care, handling and killing.
Insulin tolerance test Animals were fasted for 4 h, after which a basal blood sample was taken from the tail tip, then insulin (Humulin R, Lilly; 0.5 IU/kg body weight) was injected subcutaneously. At 40 and 90 min after insulin administration, blood was sampled. The blood glucose levels and the area under the glucose curves plotted from data collected during the insulin tolerance test (ITT) were calculated.
Oral glucose tolerance test Animals were fasted for 4 h, after which a basal blood sample was taken from the tail tip, then they were given glucose (2 g/kg body weight) oral treatment. At 30, 60 and 120 min after glucose load, blood was sampled. The blood glucose levels and the area under the glucose curves generated from data collected during the oral glucose tolerance test (OGTT) were calculated.
Biochemical analysis Serum glucose levels were assayed using the glucose oxidase method. Triglyceride (TG) levels were determined using the colorimetric method with commercial kits (Zhong Sheng Bei Kong Bio-Technology and Science, Beijing, China). Serum insulin levels were assayed with radioimmunoassay kits (Chinese Institute of Atomic Energy, Beijing, China). The insulin sensitivity index (ISI) was calculated by using the values of glucose and insulin.
Statistical analysis The values presented are expressed as mean±SD. Statistical analyses were carried out using ANOVA. P<0.05 was considered to be statistically significant.
Results
In vitro studies
Reporter gene assays The selectivity and potency of compound I, rosiglitazone and WY-14643 on human PPARα and PPARγ were determined in reporter gene assays in which the ligand-binding domain of the nuclear receptors was the primary target. The fold induction of the solvent was defined as 1. The concentration-effect curves (Figure 1) showed that compound I clearly activated both PPARα and PPARγ.
Pharmacological studies
Effect of compound I on insulin tolerance test in KKAy mice The ITT was performed on the 10th day of treatment. The results of the ITT showed that insulin resistance had developed significantly in KKAy mice (Figure 2). Treatment with rosiglitazone markedly decreased glucose levels at every time point and the area under the curve (AUC). Plasma glucose levels in the KKAy mice treated with AZ242 and compound I at 7 μmol·kg-1·d-1 were significantly reduced compared with the control group at all time points, indicating that insulin sensitivity was improved.
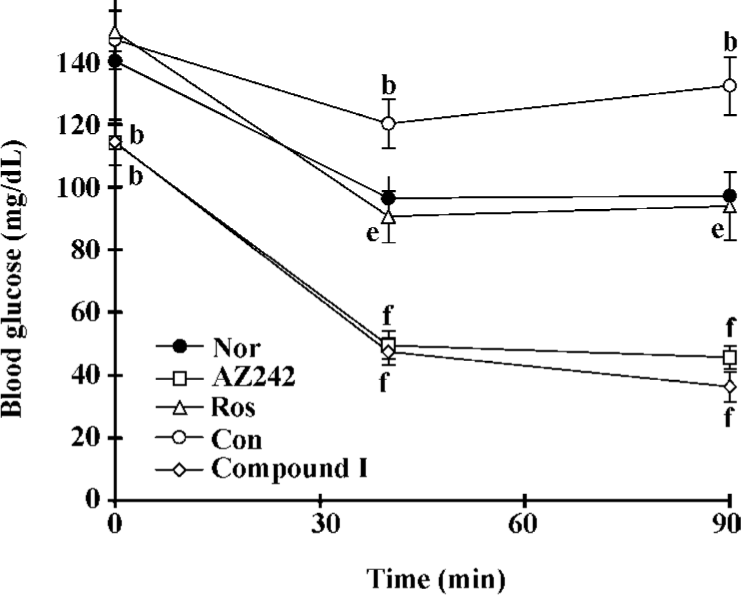
Effect of compound I on oral glucose tolerance test in KKAy mice The OGTT was performed on the 14th day of treatment. The glucose responses during the OGTT are shown in Figure 3. The OGTT results revealed that KKAy mice had impaired glucose tolerance compared with the normal group. Treatment with rosiglitazone markedly decreased glucose levels at every time point. Plasma glucose levels in the KKAy mice treated with AZ242 and compound I at 7 μmol/kg per d were significantly lower than the controls at all time points. The AUC (0–120 min) for the glucose response during the OGTT in the treatment groups was significantly less than the control. These results suggest that compound I improves impaired glucose tolerance in KKAy mice.
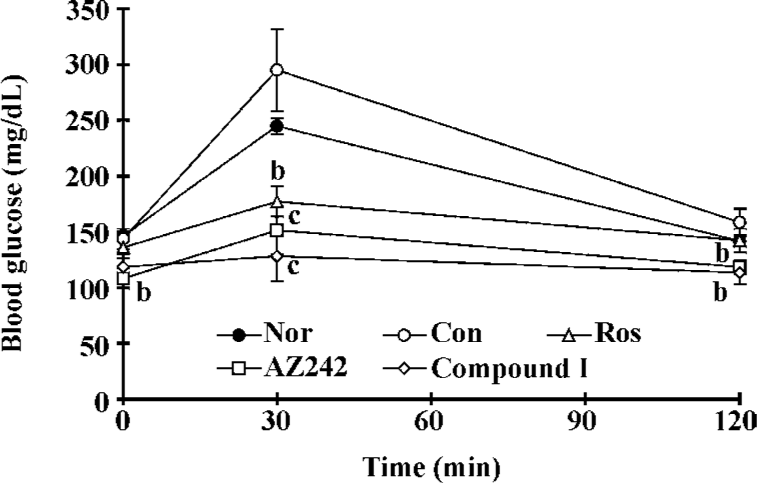
Effect of compound I on plasma triglycerides, fasting blood insulin, and ISI in KKAy mice KKAy mice had significantly elevated levels of plasma triglycerides and insulin compared with normal mice (Table 1). Reduced ISI [1/(fasting blood glucose×fasting blood insulin)] values revealed that insulin resistance developed in KKAy mice. Treatment with rosiglitazone improved insulin sensitivity, and treatment with AZ242 and compound I significantly lowered fasting glucose, plasma triglyceride and insulin levels. ISI values in the KKAy mice were significantly higher than those seen in the control group.
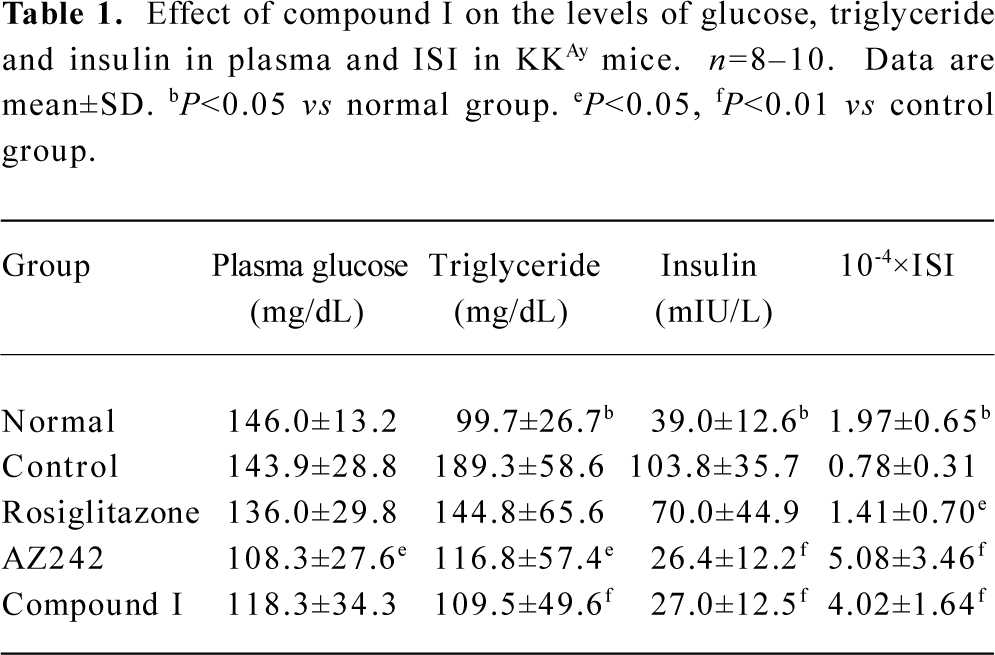
Full table
Discussion
In the present study, genetically insulin-resistant obese KKAy mice were used which are known to display marked hyperglycemia, hyperinsulinemia, and elevated triglyceride levels[16], features common to human type 2 diabetes. The PPAR are dietary lipid sensors that regulate fatty acid and carbohydrate metabolism. The hypolipidemic effects of fibrate drugs and the therapeutic benefits of thiazolidinedione drugs are due to their activation of PPARα and PPARγ, respectively. Our experiments using an in vitro reporter assay indicated that compound I activated PPARα- and PPARγ-mediated transcription, suggesting that compound I may modulate reactions that are regulated by these transcriptional activators.
Metabolic syndrome, which is particularly relevant to insulin resistance, is characterized by glucose intolerance, hyperinsulinemia, dyslipidemia, and hypertension and is frequently associated with visceral obesity. The clustering of these multiple cardiovascular risk factors increases the risk of developing atherosclerotic vascular disease. Pharmacological treatment of this syndrome aims to reduce insulin resistance and other risk factors by modulating PPAR, nuclear hormone receptors that play a key role in regulating energy metabolism. Fibrate drugs, which act as ligands for PPARα, and thiazolidinedione drugs, which are ligands for PPARγ, are often used to treat hyperlipidemia and hyperglycemia, respectively. Combination therapy using these medications is an attractive option for the treatment of obese type 2 diabetics[17,18]. Compounds that have dual agonistic activity on both of these receptors have been shown to improve insulin sensitivity and dyslipidemia in obese diabetic animals[19,20]. In the present study, when KKAy mice, used as models of noninsulin-dependent diabetes, were treated with compound I and AZ242, their insulin resistance and hyperlipidemia were improved. The effect noted was similar to that observed following treatment with pioglitazone, a thiazolidinedione drug.
In conclusion, our results imply that compound I activates both PPARα and PPARγ, and improves insulin sensitivity and lipid metabolism. The simultaneous activation of PPARα and PPARγ by compound I may be an effective approach for the treatment of metabolic syndrome, in which glucose and lipid metabolism are both impaired.
References
- Port DJ, Schwartz MW. Diabetes complications: why is glucose potentially toxic? Science 1996;27:699-700.
- Ding SY, Shen ZF, Chen YT, Sun SJ, Quan Liu, Xie MZ. Pioglitazone can ameliorate insulin resistance in low-dose streptozotocin and high sucrose-fat diet induced obese rats. Acta Pharmacol Sin 2005;26:575-80.
- Berger J, David E. The mechanisms of action of PPARs. Annu Rev Med 2002;53:409-35.
- Barry GS, William JH. Recent advances in peroxisome proliferator-activated receptor science. Curr Med Chem 2003;10:267-80.
- Torra IP, Gervois P, Staels B. Peroxisome proliferator-activated receptor alpha in metabolic disease, inflammation, atherosclerosis and aging. Curr Opin Lipidol 1999;10:151-60.
- Staels B, Dallongeville J, Auwerx J, Schoonjans K, Leitersdorf E, Fruchart JC, et al. Mechanism of action of fibrates on lipid and lipoprotein metabolism. Circulation 1998;98:2088-93.
- Chaput E, Saladin R, Silvestre M, Edgar AD. Fenofibrate and rosiglitazone lower serum triglycerides with opposing effects on body weight. Biochem Biophys Res Commun 2000;271:445-50.
- Lehmann JM, Moore LB, Smith-Oliver TA, Wilkison WO, Willson TM, Kliewer SA, et al. An antidiabetic thiazolidinedione is a high affinity ligand for peroxisome proliferators-activated receptor γ (PPARγ). J Biol Chem 1995;270:12953-6.
- Willlson TM, Cobb JE, Cowan DJ, Wiethe RW, Correa ID, Prakash SR, et al. The structure-activity relationship between peroxisome proliferator-activated receptor γ agonism and the antihyperglycemic activity of thiazolidinediones. J Med Chem 1996;39:665-8.
- Aronoff S, Rosenblatt S, Braithwaite S, Egan JW, Mathisen AL, Schneider RL, et al. Pioglitazone hydrochloride monotherapy improves glycemic control in the treatment of patients with type 2 diabetes: a 6-month randomized placebo-controlled dose-response study. Diabetes Care 2000;23:1605-11.
- Mathisen AL, Brockley MR. The relationship of HbAlc and weight in the treatment of patients with type 2 diabetes. Diabetes 2000;49 Suppl 1:A117.
- Brooks DA, Etgen GJ, Rito CJ, Shuker AJ, Dominianni SJ, Warshawsky AM, et al. Design and synthesis of 2-methyl-2-{4-[2-(5-methyl-2-aryloxazol-4-yl)ethoxy]phenoxy}propionic acids: a new class of dual PPAR α/γ agonists. J Med Chem 2001;44:2061-4.
- Desai RC, Han W, Metzger EJ, Bergman JP, Gratale DF, MacNaul KL, et al. 5-Aryl thiazolidine-2,4-diones: discovery of PPAR dual α/γ agonists as antidiabetic agents. Bioorg Med Chem Lett 2003;13:2795-8.
- Ebdrup S, Pettersson I, Rasmussen HB, Deussen HJ, Jensen AF, Mortensen SB, et al. Synthesis and biological and structural characterization of the dual-acting peroxisome proliferator-activated receptor α/γ agonist ragaglitazar. J Med Chem 2003;46:1306-17.
- Li Z, Liao C, Ko BC, Shan S, Tong EH, Yin ZH, et al. Design, synthesis, and evaluation of a new class of noncyclic 1,3-dicarbonyl compounds as PPARα selective activators. Bioorg Med Chem Lett 2004;14:3507-11.
- Iwatsuka H, Shino A, Suzuoki Z. General survey of diabetic features of yellow KK mice. Endocrinol Jpn 1970;17:23-5.
- Shimamura M, Hazato T, Ashino H, Yamamoto Y, Iwasaki E, Tobe H, et al. Inhibition of angiogenesis by humulone, a bitter acid from beer hop. Biochem Biophys Res Commun 2001;289:220-4.
- Yamamoto K, Wang J, Yamamoto S, Tobe H. Suppression of cyclooxygenase-2 gene transcription by humulon of beer hop extract studied with reference to glucocorticoid. FEBS Lett 2000;465:103-6.
- Ljung B, Bamberg K, Dahllof B, Kjellstedt A, Oakes ND, Ostling J, et al. AZ 242, a novel PPARalpha/gamma agonist with beneficial effects on insulin resistance and carbohydrate and lipid metabolism in ob/ob mice and obese Zucker rats. J Lipid Res 2002;43:1855-63.
- Murakami K, Tobe K, Ide T, Mochizuki T, Ohashi M, Akanuma Y, et al. A novel insulin sensitizer acts as a coligand for peroxisome proliferator-activated receptor-alpha (PPAR-alpha) and PPAR-gamma: effect of PPAR-alpha activation on abnormal lipid metabolism in liver of Zucker fatty rats. Diabetes 1998;47:1841-7.