Growth-promoting activity of Hominis Placenta extract on regenerating sciatic nerve1
Introduction
Nerve fibers in the peripheral nervous system (PNS), unlike those in the central nervous system (CNS), regrow toward their original target after injury, and are capable of functional regeneration[1]. This difference in regeneration capability between PNS and CNS is attributed to both intrinsic neuronal determinants and extrinsic environmental factors. For instance, axonal growth-associated protein GAP-43 is highly induced by peripheral nerve injury, but not by axon tract injuries in the spinal cord[2]. Overexpression of GAP-43 and cytoskeleton-associated protein CAP-23 in transgenic mice was able to induce the regeneration of injured spinal cord axons, implying that the supply of peripheral regeneration factors can similarly induce axonal regeneration after spinal cord injury[3]. In addition to the intrinsic factors, differences in the nature of the molecules in the surrounding environments of CNS and PNS are critical for determining the responsiveness of injured axons. Increased propagation of Schwann cells and activated macrophages in an area of injured peripheral nerve is important for promoting axonal regrowth, whereas glial scar tissues containing chondroitin sulfate proteoglycan, EphB3 and semaphorin 3A peptidoglycan sulfate, and induced myelin components in the oligodendrocytes such as Nogo and MAG, inhibit spinal cord regeneration[4–6].
Although injured peripheral axons have a clear advantage with respect to regenerative capacity compared with CNS axons, peripheral nerve repair leading to functional recovery is seldom perfect. After axotomy, regrowing axons need to be correctly guided into the growth environment of the distal nerve stump, which is primarily provided by activated Schwann cells. Furthermore, targeting is usually far from perfect, especially if the injury requires regeneration over long distances. Al-Majed et al have reported that the correct targeting of sensory and motor neurons after femoral nerve transection was much improved by electrical stimulation[7]. Thus, the whole process, including the removal of nerve debris following Wallerian degeneration, formation of whole basal lamina/Schwann cell tubes (called the band of Büngner), and growth cone elongation are equally important for successful functional recovery.
Most studies on axonal regeneration have been primarily focused on axonal regrowth and elucidating molecular factors, and a majority of molecular factors so far reported are endogenous molecules. Despite the many possibilities regarding the roles of exogenous molecular factors in axonal regeneration, little research has been carried out in this field. Oriental medicinal drugs have a broad spectrum of clinical uses, including for the cure of cardiovascular and nervous system diseases, and some of them have been found to have specific effects on brain diseases such as stroke and diabetic neuropathy[8,9]. A water extract of Hominis Placenta (HP) has been used in oriental medicine for the treatment of diseases of the brain, kidney, and other organs, and to supplement “vital essences”[10]. Here, we report that HP had a growth-promoting activity for injured sciatic axons in vivo and in cultured dorsal root ganglia (DRG) sensory neurons.
Materials and methods
Drug preparation Dried human placenta (HP) was obtained from Daejeon University Oriental Medicine Hospital (Daejeon, Korea). HP is approved for in vivo injection by the Korean Food and Drug Administration (KFDA), and HP extract was prepared as described elsewhere[21]. Briefly, dried HP was suspended in 2 L of water, heat-extracted for 3 h, and filtered 3 times. The filtered fluid was distilled using a rotary vacuum evaporator. Concentrated solutions were frozen at -70 oC for 4 h, and freeze-dried for 24 h. The product was kept at 4 oC, and dissolved in water. The stock solution was stored at -20 oC and diluted with a physiological saline solution before use.
Sciatic nerve surgery Sprague-Dawley rats (8 weeks old, male) were housed individually in cages in a temperature-controlled room with a 12-h light and dark cycle. A total of 56 rats were used in the present experiment. Animals were anesthetized by intraperitoneally (ip) injecting a mixture of ketamine (80 mg/kg) and xylazine (5 mg/kg), and the sciatic nerve was exposed and crushed with a pair of forceps held tightly for 30 s twice at 1 min interval[11]. For drug administration, the Hamilton microsyringe (600 series, gauge 22s; Hamilton, USA) attached to a microinjection apparatus (Stoelting, USA) was placed onto the injury site immediately after the sciatic nerve was crushed, and 5 μL of HP solution or an equivalent volume of saline was injected slowly for 5 min. The dose-dependent effects of HP on axonal regeneration were examined by injecting 5 μL of HP solutions of different concentrations (2, 10, and 40 μg/mL), corresponding to dosages of 50, 250, and 1000 μg/kg for rats with a body weight of 200 g, respectively. Animals recovered from anesthesia and were killed 7 d later. Before being killed, animals were deeply anesthetized with a mixture of ketamine and xylazine, and the sciatic nerves were dissected, immediately frozen, and kept at -75 oC until use. For the purposes of immunofluorescence staining, the sciatic nerve was prepared by dividing it into the proximal stump (a 5-mm segment proximal to the injury site) and the distal stump (5 mm segment distal to the injury site).
Histology and immunofluorescence staining Nerve segments were embedded into embedding media (Histo Prep, Fisher Scientific, USA) and frozen at -20 oC. Longitudinal or transverse sections (20 mm thickness) were cut on a cryostat and mounted on glass slides that electrostatically attract frozen and formalin-fixed tissue sections (Superfrost Plus, Fisher Scientific). For double immunofluorescence staining, sections were fixed with 4% paraformaldehyde and 4% sucrose in phosphate-buffered saline (PBS) at room temperature for 40 min, permeabilized with 0.5% nonidet P-40 in PBS, and blocked with 2.5% horse serum and 2.5% bovine serum albumin for 4 h at room temperature. Sections were incubated with anti-neurofilament-200 antibody (NF-200, clone no. N52, mouse monoclonal, diluted 1:200; Sigma, USA), anti-GAP-43 antibody (H-100, rabbit polyclonal, diluted 1:200; Santa Cruz Biotech, USA), anti-βIII-tubulin antibody (TUJ1, rabbit polyclonal, diluted 1:200; Covance, USA), anti-S100β antibody (rabbit polyclonal, diluted 1:200; Dako, Denmark), anti-Cdc2 antibody (p34, mouse monoclonal, diluted 1:200; Santa Cruz Biotech), then incubated with fluorescein-goat anti-mouse (diluted 1:400; Molecular Probes, USA) or rhodamine-goat anti-rabbit secondary antibodies (diluted 1:200, Molecular Probes) in 2.5% horse serum and 2.5% bovine serum albumin for 1 h at room temperature and coverslipped with gelatin mount medium. We always included the control sections treated with secondary antibody alone, which usually did not have any visible images. In cases when the nonspecific signals were high, no data from those experiments were analyzed further. Sections were viewed with a Nikon fluorescence microscope and the images were captured using a Nikon digital camera (Nikon). To minimize experimental variation, sections from different animals in an experiment were always treated with the same solutions throughout the whole immunostaining process, and the images for a given experiment were captured after the same exposure time using Nikon ACT-1 software. Adobe Photoshop (version 5.5) was used to process the images. For all the sections from the individual experiments, the merged images were produced by the layer blending mode options of the Photoshop program.
Western blot analysis Nerve segments were washed with ice-cold PBS, and sonicated under 50–200 mL of triton lysis buffer [20 mmol/L Tris, pH 7.4, 137 mmol/L NaCl, 25 mmol/L β-glycerophosphate, pH 7.14, 2 mmol/L sodium pyrophosphate, 2 mmol/L ethylenediamine tetraacetic acid (EDTA), 1 mmol/L Na3VO4, 1% Triton X-100, 10% glycerol, 5 mg/mL leupeptin, 5 mg/mL aprotinin, 3 mmol/L benzamidine, 0.5 mmol/L DTT, 1 mmol/L PMSF]. Ten micrograms of proteins were used for Western blotting analysis using anti-GAP-43 antibody (H-100, rabbit polyclonal, Santa Cruz Biotech) or anti-Cdc2 antibody (p34, mouse monoclonal, Santa Cruz Biotech). Electrophoresis and western blotting were performed as described previously[11]. Primary and secondary antibodies were diluted to 1:100 and 1:10 000, respectively, and used as recommended by the manufacturers. To confirm the immunoreaction specificity of the antibodies to GAP-43 and Cdc2, a control experiment was performed by supplying an excess of purified GAP-43 and Cdc2 proteins (Calbiochem, USA) as antigens, using the protocol provided by the manufacturer (Acris Antibodies, Germany). Briefly, 2 μg of antibody was mixed with 20 μg of antigen protein in 100 μL of PBS at 37 oC for 2 h, and centrifuged for 15 min at 4 oC at 10 000 r/min. Then, 50 μL of the bottom phase containing immune complexes in the tube was taken and mixed with 0.1% triton X-100 in PBS for western blot analysis. Quantitative analysis of protein levels in the autoradiographic images was determined by using the i-Solution software package (Image and Microscope Technology, USA).
Retrograde tracing of motor neurons in the spinal cord The sciatic nerves of rats anesthetized with ketamine and xylazine were exposed, and DiI (5 μL of a 3% solution in dimethylsulfoxide; Molecular Probes) was applied to the portion of the sciatic nerve 10 mm distal to the injury site by using a microsyringe. The incision was sutured, and the animals were returned to their cages after recovering from the anesthesia. Forty eight hours later, animals were killed and all the sections collected (20 μm thickness) were used to count diI-labeled motor neurons observed at the T11–12 levels. The mean number of total labeled cells in individual animals was compared among groups by using Student’s t-test. Cell counting analysis was conducted with the examiner blinded to the experimental treatment conditions.
Primary DRG sensory neuron culture Glass coverslips were precoated with a mixture of poly-L-ornithine (0.1 mg/mL; Sigma) and laminin (0.02 mg/mL; Collaborative Research, USA) in a 37 oC, 5% CO2 incubator. L4 and L5 DRG were removed from adult male rats, and placed in ice-cold Dulbecco’s modified Eagle’s medium (DMEM; Gibco, USA). The ganglia were treated with DMEM containing type XI collagenase (2500 U/mL; Sigma) for 90 min at 37 oC. Tissues were then washed with DMEM medium and centrifuged at 800 r/min for 1 min to remove the supernatant. After one more wash, cells were suspended in DMEM, dissociated gently with 16–20 passages through a flamed Pasteur pipette, and centrifuged at 800 r/min for 1 min to remove the supernatant. Cells were then treated with DMEM containing type SII trypsin (0.5 mg/mL) for 10 min followed by DMEM containing trypsin inhibitor (100 mg/mL), EDTA (1 mmol/L) and DNase I (80 mg/mL) for 5 min. After cells were washed with culture medium [DMEM containing 5% heat-inactivated fetal bovine serum (FBS; Gibco), 5% horse serum, 2 mmol/L glutamine and 1% penicillin-streptomycin], 800–1200 neurons were plated onto 12 mm round coverslips and cultured for 12 h in a 37 oC, 5% CO2 incubator with fresh culture medium. DRG neurons were treated with HP (50 μg/mL or 200 μg/mL) or saline vehicle and cultured for 24–48 h. Cells grown on the coverslips were fixed with 4% paraformaldehyde/4% sucrose solution for 45 min at room temperature and used for immunofluorescence staining. Images of immunostained cells were captured on a digital camera, and neurite arborization and length were quantitatively assessed by using the i-Solution software package (Image and Microscope Technology).
Results
Effect of HP treatment on GAP-43 protein levels in regenerating sciatic nerves Crush injury induced moderate levels of GAP-43 signaling in both the proximal and distal stumps compared with intact animals (Figure 1A). Treatment with HP at concentrations of 50 μg/kg, 250 μg/kg and 1 mg/kg enhanced GAP-43 protein signaling in both the proximal and distal segments of the injured sciatic nerves in a dose-dependent manner (Figure 1A, 1B); that is, GAP-43 signals in the injured sciatic nerve with a dosage of 250 μg/kg of HP were stronger than those in nerves treated with 50 μg/kg HP, and reached similar levels as those achieved in nerves treated with 1 mg/kg. Thus, we used an HP dose of 250 μg/kg for the remaining in vivo experiment in the present study. HP administration (250 μg/kg) into the intact nerve did not induce any GAP-43 protein (Figure 1A). Immunostaining of the same nerve sections with the neurofilament protein NF-200, a neuronal marker, revealed that signals for the GAP-43 protein mostly overlapped with those for NF-200 (shown in yellow in the merged image; Figure 1C).
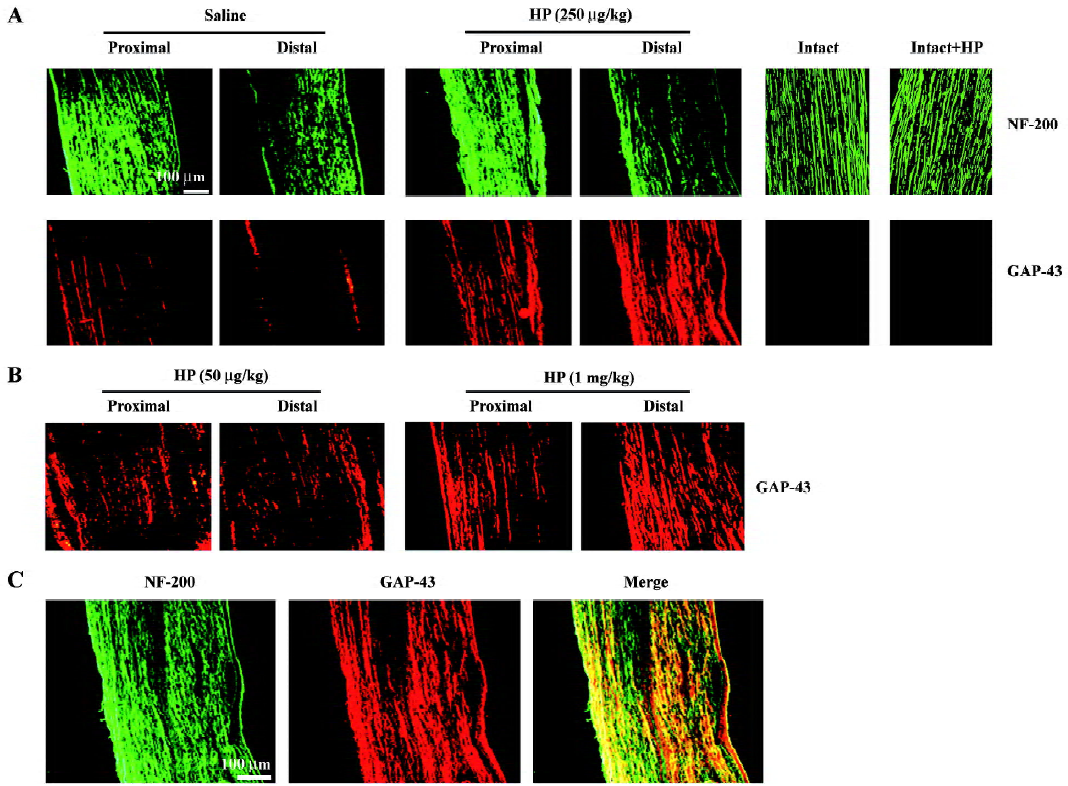
To determine the GAP-43 expression levels in crushed nerves after HP treatment, proteins in the sciatic nerves were analyzed by Western blotting. GAP-43 protein was induced in the injured sciatic nerves, particularly in the proximal stump (Figure 2A, 2B). HP treatment further increased the amount of GAP-43 protein in the distal as well as the proximal stump of the sciatic nerves. Western blotting analysis of nerve tissues after preincubation of the anti-GAP-43 antibody with excess GAP-43 protein completely eliminated GAP-43 signals, indicating the reaction specificity between the anti-GAP-43 antibody and the protein samples (Figure 2C).
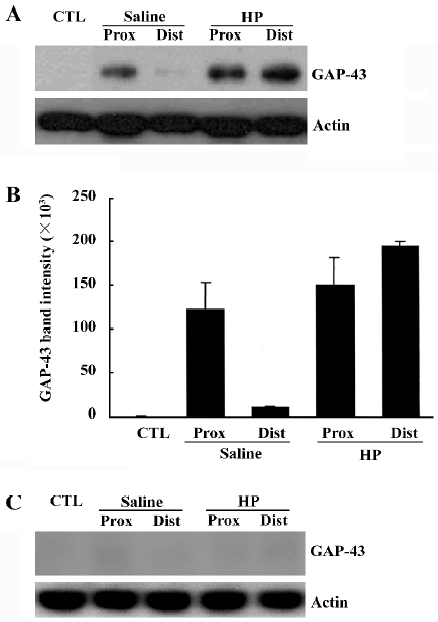
Upregulation of Cdc2 protein levels in the injured sciatic nerve caused by HP treatment We recently found that cell division cycle 2 (Cdc2) kinase, a key regulatory protein for the progression from G2 to M phase in the cell cycle[12], is strongly induced in injured nerves[11,13], and is required for axonal regeneration (unpublished data). In the present study, we examined whether HP regulates Cdc2 protein levels in the regenerating sciatic nerve. Cdc2 protein was not detected in the uninjured sciatic nerve, but there was a strong induction in the distal, but not in the proximal, stump of the nerves 7 d after injury (saline control in Figure 3A, 3B). HP treatment further increased the levels of Cdc2 protein in the distal stump of the injured sciatic nerve. Western blotting analysis of nerve tissues after preincubation of anti-Cdc2 antibody with excess Cdc2 protein completely inhibited Cdc2 signals, indicating the reaction specificity between the anti-Cdc2 antibody and protein samples (Figure 3C).
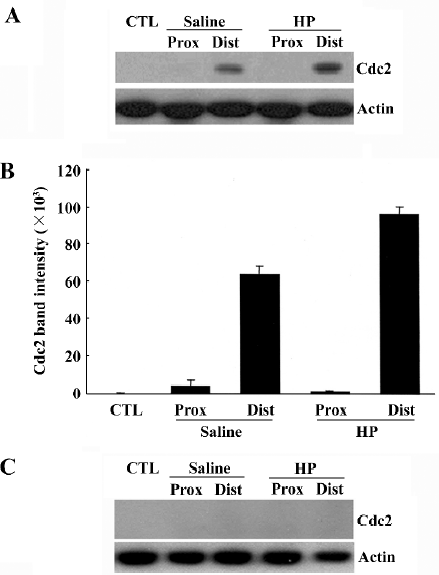
Immunofluorescence staining also indicated an increased Cdc2 protein signal in the injured nerves with HP treatment (Figure 4A). To localize induced Cdc2 protein signals in the nerve tissues, double immunofluorescence staining was performed using transverse sections of the sciatic nerve 3 mm distal to the injury site. S100β is the myelin protein that is selectively expressed in Schwann cells. Induced Cdc2 signal in the injured sciatic nerve with HP treatment mostly overlapped with the S100β signal, but not the axon-specific βIII-tubulin signals (Figure 4B). Next, we examined the cell numbers in the areas of the nerves undergoing axonal regenera-tion. The non-neuronal cell population in the distal stump was visualized by nuclear staining of longitudinal nerve sections with the Hoechst 33258 dye. Nuclear counts were increased in the injured nerves relative to the uninjured control group, and further increases were observed with HP treatment in the corresponding area of the sciatic nerve (Figure 4C). These data, along with increased Cdc2 protein expression, suggest that HP treatment causes increased Schwann cell proliferation in injured sciatic nerves.
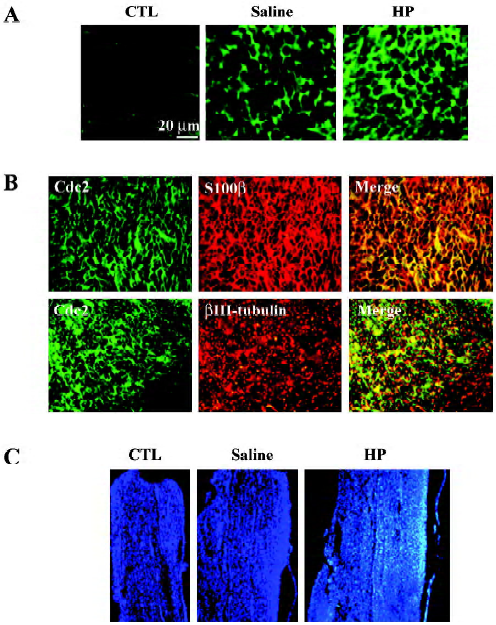
Improved axonal regeneration caused by HP treatment In an animal group with no injury, injection of diI into the nerve labeled motor neuron cell bodies (Figure 5A). In a group with sciatic nerve injury that was injected with saline, the number of diI-labeled motor neurons was much less compared with the control group, suggesting that the majority of injured nerve fibers did not regenerate to a position 10 mm distal to the injury site by d 7 post crush. In the injured nerves treated with HP, the number of diI-labeled neurons was enhanced, reaching similar levels as in the control. To confirm the microscopic observations, all diI-labeled cells from individual sections were summed, and a numerical comparison was made. As shown in Figure 5B, the number of diI-labeled cells in the HP-treated injured neurons was significantly greater relative to the number in the saline-treated injury group. These data suggest that HP is effective in promoting sciatic nerve fiber regeneration after crush injury.
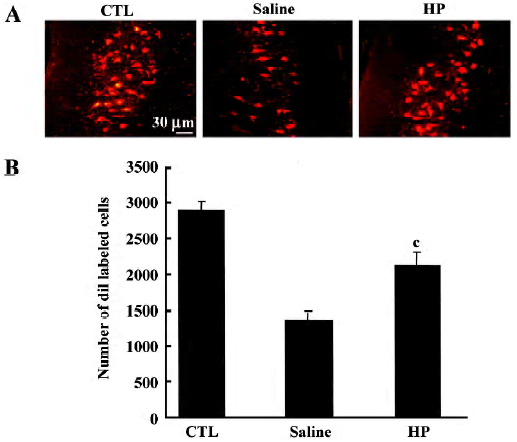
Enhanced neurite outgrowth of DRG sensory neurons caused by HP treatment Neurite outgrowth in the group with sciatic nerve injury (designated the “preconditioned” group) involved elongation and arborization to a much greater extent compared with the non-injury control, indicating that the lesion signals in response to nerve crush induce neurite outgrowth of DRG sensory neurons in culture (data not shown). In order to examine the effect of HP on axonal regeneration of DRG sensory neurons in terms of neurite outgrowth, cells were exposed to HP for 24 h. Neurons treated with 50 μg/mL HP had significantly longer neurite outgrowth processes and branches compared with the saline-treated group (Figure 6A–C). When HP concentration was increased to 200 μg/mL, there were further increase in neurite out-growth.
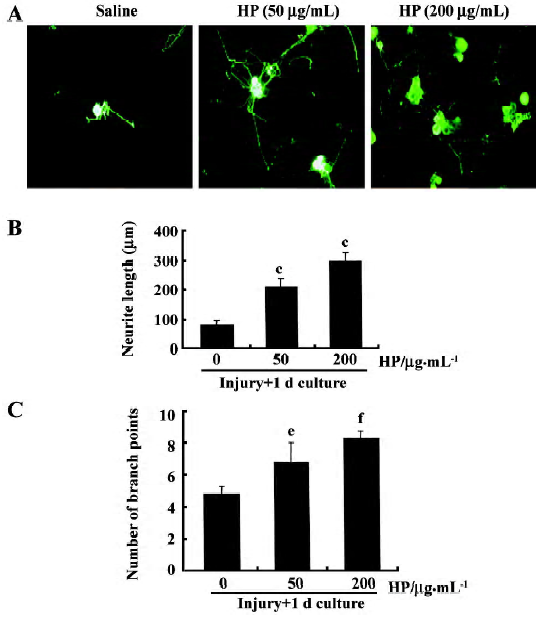
Discussion
The present study provides compelling evidence that HP is a biologically active drug that promotes the recovery of impaired nerves. Local administration of HP upregulated GAP-43 and Cdc2 protein levels in regenerating nerves. Observations of regenerating axons in vivo using retrograde tracers and of in vitro regeneration potential using preconditioned DRG sensory neurons indicated that there was enhanced axonal re-growth in HP-treated nerves compared with non-treated controls.
Axonal growth-associated protein GAP-43 is selectively expressed in the neural system and is highly localized in the axons. Previous studies have shown that increased synthesis of GAP-43 protein is closely linked with axonal regeneration processes as an intrinsic determinant for axonal elongation in both peripheral and spinal cord axons[3,14]. In the current study, we screened several different drugs used in oriental medicine, all of which are used clinically for the treatment of nervous system diseases. Of all drugs examined, the induction levels of GAP-43 protein were highest in the sciatic nerves treated with HP at the injury site (data not shown). GAP-43 protein induced by HP treatment of the sciatic nerve mostly colocalized with axon-specific protein NF-200 signals, and was strongly induced in the distal stump where active axonal re-growth occurs following Wallerian degeneration[1,4].
Our data also indicate strong induction of the Cdc2 protein in the distal stump of the regenerating sciatic nerves with HP treatment. Cdc2 protein, a prototypical cyclin-dependent kinase, regulates the mitotic phase of the cell cycle[15]. Recent studies have also demonstrated the involvement of Cdc2 in cell migration processes[16] and in the process of neuronal apoptosis via the activation of proapoptotic protein Bad[17]. We have recently found strong induction of the Cdc2 protein in regenerating nerves such as facial or sciatic nerves[11,13]. The induced Cdc2 protein signals mostly overlapped with the S100β protein signals, indicating predominant Cdc2 expression in peripheral non-neuronal cells such as Schwann cells, although the possible induction and role of Cdc2 protein in the regenerating axon itself cannot be completely ruled out. Interestingly, HP-treated injured nerves had increased numbers of Hoechst-stained nuclei in the injured sciatic nerves. Because Schwann cells are important for guiding growing axons toward their target muscles or sensory organs, it is possible that HP might act as a positive regulator for the growth-promoting actions of Schwann cells via increased Cdc2 activity. Recent characterization of Cdc2 substrates in vivo has suggested diverse molecular interactions for cell-cycle regulation and other cell functions[18]. Experiments using a Cdc2 kinase inhibitor to regulate Schwann cell proliferation would be informative regarding the role of the Cdc2 protein in the axonal regeneration process.
Our data further demonstrate that HP is involved in axonal regeneration after injury. Measurement of regenerating motor neuron axons in vivo by using a retrograde tracer showed increased numbers of regenerating motor neurons in the spinal cord in animals treated with HP. We also examined the effects of HP treatment on the neurite outgrowth of cultured DRG sensory neurons. DRG sensory neurons are useful for studying regeneration in vitro because of their anatomical structure, which extends to both the peripheral and central nervous systems[19]. DRG sensory neurons have increased neurite outgrowth potential when sciatic nerve injury is inflicted a week before DRG culture[20]. The addition of HP at concentrations of 50–200 μg/mL induced increased neurite outgrowth of DRG sensory neurons, suggesting that HP has a growth-promoting activity in regenerating DRG sensory axons.
Although HP has been used for a long period of time in oriental medicine for the cure of physiological abnormalities in human organs, the explanations of its effects have tended to be descriptive rather than quantitative. HP treatment has been reported to have an alleviating effect on arthritic symptoms in a rat model when treatment was accompanied by acupuncture therapy[21]. HP treatment has also been reported to induce hematopoiesis[22]. HP preparation in clinical oriental medicine involves several processes, including drying, boiling, and freeze-drying human placenta, and thus the major biologically active proteins or other macromolecules in the tissues would be degraded or inactivated, and small molecules such as estradiol, progesterone, and several monosaccharides and amino acids would remain as the active components[10]. According to the present data, HP exerted an axonal growth promotion effect at multiple levels, including induction of Cdc2 and GAP-43 proteins, sciatic nerve elongation, and neurite outgrowth of DRG sensory neurons, thus it is likely that more than one chemical component of HP might act on the axonal regeneration processes.
Despite the growing body of evidence regarding the role of diverse molecular factors in axonal regeneration in both the PNS and CNS, there are only a few reports on the effects of herbal drugs on axonal regeneration. Injection of a buyang huanwu decoction (Radix hedysari) into injured sciatic nerves has been reported to promote axonal regeneration[23,24]. Ginsenoside Rb1 has also been shown to be effective for peripheral nerve regeneration and for the survival of injured spinal cord neurons[25,26]. Administration of herbal mixtures is reported to enhance the survival and regeneration of axoto-mized retinal ganglion cells[27]. Although these studies suggest that herbal drugs may be potentially useful for axonal regeneration, the molecular mechanisms underlying their actions remain to be investigated. An initial step toward this goal would be to identify the major chemical components in the herbal drugs and investigate their effects at the molecular level. In this respect, our findings regarding the induction of GAP-43 and Cdc2 protein levels by HP treatment in regenerating nerves are significant because the study provides important parameters for assessing regeneration in a quantitative way. Future studies to characterize and examine the chemical components of HP would be useful to elucidate molecular mechanisms by which it exerts its effects, and to develop drug therapies for functional regeneration.
References
- Fawcett JW, Keynes RJ. Peripheral nerve regeneration. Annu Rev Neurosci 1990;13:43-60.
- Schreyer DJ, Skene JH. Injury-associated induction of GAP-43 expression displays axon branch specificity in rat dorsal root ganglion neurons. J Neurobiol 1993;24:959-70.
- Bomze HM, Bulsara KR, Iskandar BJ, Caroni P, Skene JH. Spinal axon regeneration evoked by replacing two growth cone proteins in adult neurons. Nat Neurosci 2001;4:38-43.
- Ide C. Peripheral nerve regeneration. Neurosci Res 1996;25:101-21.
- Behar O, Mizuno K, Neumann S, Woolf CJ. Putting the spinal cord together again. Neuron 2000;26:291-3.
- Davies SJ, Fitch MT, Memberg SP, Hall AK, Raisman G., Silver J. Regeneration of adult axons in white matter tracts of the central nervous system. Nature 1997;390:680-3.
- Al-Majed AA, Neumann CM, Brushart TM, Gordon T. Brief electrical stimulation promotes the speed and accuracy of motor axonal regeneration. J Neurosci 2000;20:2602-8.
- Gong X, Sucher NJ. Stroke therapy in traditional Chinese medicine (TCM): prospects for drug discovery and development. Trends Pharmacol Sci 1999;20:191-6.
- Heng X, Zhang F. Advances in treatment of diabetic neuropathy by traditional Chinese medicine. J Tradit Chin Med 1998;18:146-52. Chinese..
- Liu G. Chinese herbal medicine. Beijing: Hua Xia Medical Publishing; 2001.
- Namgung U, Choi BH, Park S, Lee JU, Seo HS, Suh BC, et al. Activation of cyclin-dependent kinase 5 is involved in axonal regeneration. Mol Cell Neurosci 2004;25:422-32.
- Dorée M, Galas S. The cyclin-dependent protein kinases and the control of cell division. FASEB J 1994;8:1114-21.
- Seo TB, Han IS, You BG, Jeong IG, Yoon JW, Namgung U. Induction of Cdc2 protein by sciatic nerve injury and up-regulation by exercise in rat. Society for Neurosci Abstr 2004; 618.8.
- Benowitz LI, Routtenberg A. GAP-43: an intrinsic determinant of neuronal development and plasticity. Trends Neurosci 1997;20:84-91.
- Pines J. Four-dimensional control of the cell cycle. Nat Cell Biol 1999;1:E73-9.
- Manes T, Zheng DQ, Tognin S, Woodard AS, Marchisio PC, Languino LR. Alpha(v)beta3 integrin expression up-regulates cdc2, which modulates cell migration. J Cell Biol 2003;161:817-26.
- Konishi Y, Lehtinen M, Donovan N, Bonni A. Cdc2 phosphorylation of BAD links the cell cycle to the cell death machinery. Mol Cell 2002;9:1005-16.
- Ubersax JA, Woodbury EL, Quang PN, Paraz M, Blethrow JD, Shah K, et al. Targets of the cyclin-dependent kinase Cdk1. Nature 2003;425:859-64.
- Richardson PM, Issa VM. Peripheral injury enhances central regeneration of primary sensory neurones. Nature 1984;309:791-3.
- Smith DS, Skene JH. A transcription-dependent switch controls competence of adult neurons for distinct modes of axon growth. J Neurosci 1997;17:646-58.
- Yeom MJ, Lee HC, Kim GH, Shim I, Lee HJ, Hahm DH. Therapeutic effects of Hominis placenta injection into an acupuncture point on the inflammatory responses in subchondral bone region of adjuvant-induced polyarthritic rat. Biol Pharm Bull 2003;26:1472-7.
- Son CG, Han SH, Cho JH, Shin JW, Cho CH, Lee YW, et al. Induction of hemopoiesis by saenghyuldan, a mixture of Ginseng radix, Paeoniae radix alba, and Hominis placenta extracts. Acta Pharmacol Sin 2003;24:120-6.
- Xu H, Jiang B, Zhang D, Fu Z, Zhang H. Compound injection of radix Hedysari to promote peripheral nerve regeneration in rats. Chin J Traumatol 2002;5:107-11.
- Cheng YS, Cheng WC, Yao CH, Hsieh CL, Lin JG, Lai TY, et al. Effects of buyang huanwu decoction on peripheral nerve regeneration using silicone rubber chambers. Am J Chin Med 2001;29:423-32.
- Chen YS, Wu CH, Yao CH, Chen CT. Ginsenoside Rb1 enhances peripheral nerve regeneration across wide gaps in silicone rubber chambers. Int J Artif Organs 2002;25:1103-8.
- Liao B, Newmark H, Zhou R. Neuroprotective effects of ginseng total saponin and ginsenosides Rb1 and Rg1 on spinal cord neurons in vitro. Exp Neurol 2002;173:224-34.
- Cheung ZH, So KF, Lu Q, Yip HK, Wu W, Shan JJ, et al. Enhanced survival and regeneration of axotomized retinal ganglion cells by a mixture of herbal extracts. J Neurotrauma 2002;19:369-78.