Cardioprotective effects of ghrelin and des-octanoyl ghrelin on myocardial injury induced by isoproterenol in rats1
Introduction
Ghrelin, a novel 28-amino acid peptide principally released from the stomach, has been identified as an endogenous ligand for the growth hormone secretagogue receptor (GHSR)[1]. GHSR-1a, a G protein-coupled receptor mainly expressed in the pituitary and hypothalamus, is thought to mediate the endocrine actions of ghrelin, including its potent growth hormone (GH)-secreting effects[2]. Ghrelin mainly circulates in 2 different forms: octanoyl and des-octanoyl ghrelin. Although ghrelin is essentially a gastro-derived hormone, it has been found to be expressed ubiquitously and circulated in plasma at a concentration of approximately 100 pmol/L in healthy humans[3]. Recently, growing evidence has indicated that cardiovascular tissue is rich in ghrelin receptors, and that its mRNA is expressed abundantly in human myocardial cells, and rat ventricles, atria, aorta, coronary arteries, carotid arteries, the endocardium and vena cava[4], which confirms that the cardiovascular system is a target for ghrelin. In addition, ghrelin is an important autocrine/paracrine factor of cardiovascular tissues and has various cardiovascular effects, including increased myocardial contractility and vasodilatation, and anti-inflammation[5,6]. Our previous work showed that ghrelin was an endogenous cardioprotective factor in isolated rat hearts with ischemia/reperfusion injury, ghrelin perfusion enhanced coronary flow, ameliorated cardiac function suppression and decreased myocardial oxygen consumption[7]; in rats with heart failure induced by sepsis, ghrelin improved cardiac function and hemodyna-mics[8]; and in rats with acute myocardial necrosis induced by isoproterenol (ISO; 40 mg/kg per d, for 2 d) ghrelin administration significantly protected the heart from ischemic injury and lipid peroxidation damage[9]. However, the cardioprotective mechanism of ghrelin is still unclear.
From past studies, the effect of ghrelin was thought to depend entirely on its acylation and subsequent interaction with GHSR to promote the synthesis and secretion of GH. Recent investigations have revealed that besides having a stimulatory effect on GH secretion, ghrelin possesses a variety of effects through GH-independent mechanisms. Tsubota and coworkers reported that the microinjection of ghrelin into rat nucleus tractus solitarii (NTS) elicited hypotensive effects, but this was not the case upon injection into GHSR-expressing rostral ventrolateral medulla or caudal ventrolateral medulla. To elucidate the mechanism of ghrelin, they examined the cardiovascular response of rats microinjected with des-acyl ghrelin into NTS. Des-acyl ghrelin does not activate GHSR-1a and is devoid of endocrine activity. The hypotensive and bradycardic activity evoked by des-acyl ghrelin was not significantly different from that of native ghrelin. These results suggest that des-acyl ghrelin contri-butes to the regulation of cardiovascular control and that a receptor other than GHSR-1a exists in NTS. In addition, several reports have shown that ghrelin plays many roles that are independent of GH release[10–12]. However, it is unknown whether the cardioprotective role of ghrelin is absolutely or partly dependent on GH release.
In the present study, we used an ISO-induced myocardial injury model to observe the changes in expression of cardiac ghrelin and its receptor, and to investigate the therapeutic effects of ghrelin and des-octanoyl ghrelin and the mechanisms thereof.
Materials and methods
Animals and reagents All animal care and experimental protocols were complied with the Animal Management Rules of the Ministry of Health of the People’s Republic of China (document N
Preparation of animal model The ISO-induced subacute myocardial injury model was produced as described previously[13], with minor modifications. Thirty male SD rats were randomly and equally divided into 5 groups: (1) ISO group: ISO (20, 10, and 5 mg/kg) was subcutaneously injected on d 1, 2, and 3, respectively, and then 3 mg/kg for the next 7 d; (2) control group: rats were subcutaneously injected with normal saline for 10 d; (3) and (4) therapeutic groups: ISO administration was the same as in (1), and ghrelin and des-octanoyl ghrelin (100 µg/kg, twice daily), respectively, were subcutaneously injected for 10 d.
At the end of the experiment all rats were fasted overnight but had free access to water at the last administration of the drug. The rats were anesthetized with urethane (1 g/kg, ip), and a catheter filled with heparin saline (500 U/mL) was inserted into the right common carotid artery for measuring intraventricular pressure. The heart rate, maximal left-ventricular pressure development (LV dp/dtmax) and left ventricular end-diastolic pressure (LVEDP) were recorded by using Powerlab/4S (Santa Monica, CA, USA). After the hemodynamic parameters were measured, a blood sample was drawn from the left ventricle and mixed with 1 mg/mL ethylenediamine tetraacetic acid (EDTA)-2Na and 500 kIU/mL aprotinin. Serum was obtained by centrifugation at 600×g for 10 min at 4 ℃ and stored at -70 ℃. Hearts were removed and weighed. The heart apex was taken for pathological examination under hematoxylin-eosin staining. The left ventricular (LV) myocardial sample (100 mg) was acidified by adding 1 mmol/L acetic acid, boiling for 10 min and homogenizing. The tissue supernatant was stored at -70 ℃ and used for ghrelin radioimmunoassay.
Plasma lactate dehydrogenase (LDH) activity was measured on an automatic biochemistry analyzer. The content of lipid peroxidation product malondialdehyde (MDA) and conjugated diene (CD) in myocardia and plasma was determined by using the thiobarbituric acid test[14] and spectrometry[15], respectively, in duplication. The collagen content in the LV myocardium was determined by hydroxyproline assay as described previously[16].
Assay of mRNA levels of ghrelin and GHSR by RT-PCR[17] Total RNA from the LV myocardium (approximately 50 mg) was extracted by using Trizol reagent. A total of 1 µg of total RNA was reverse-transcribed into single-strand cDNA with oligo(dT) 15 primer and M-MuLV reverse transcriptase. RT-PCR was performed in a 0.2 mL tube containing 2 µL tissue cDNA, 1 µL of 5 µmol/L solutions of each S and A primer, 1 µL of 2.5 mmol/L of each dNTP mixture, 1.5 µL of 1.5 mmol/L MgCl2, 2.5 µL 10× PCR buffer, and 1.25 U Taq DNA polymerase, in a total volume of 25 µL. RT-PCR of ghrelin was as follows: after being denatured at 95 ℃ for 5 min, the solution underwent PCR at 94 ℃ for 30 s, 55 ℃ for 30 s, and 72 ℃ for 40 s for 30 cycles. RT-PCR of GHSR was as follows: after being denatured at 95 ℃ for 5 min, the solution underwent PCR at 94 ℃ for 30 s, 58 ℃ for 30 s, and 72 ℃ for 40 s for 30 cycles. The 6 µL PCR product was separated on a 1.5% agarose gel and stained with ethidium bromide. The optical density of the band of ghrelin mRNA (374 bp) and GHSR mRNA (321 bp) was measured by use of the Gel Documentation System (Bio-Rad, Hercules, CA, USA). The PCR products were amplified again at 94 ℃ for 30 s, 55 ℃ for 30 s, and 72 ℃for 30 s for 20 cycles with the rat β-actin primers β-actin-S and β-actin-A. The optical density of the β-actin mRNA band (291 bp) was measured. The ratio of ghrelin and GHSR mRNA to β-actin mRNA was considered the relative amount of ghrelin and GHSR mRNA, respectively.
Radioimmunoassay of ghrelin and GH Plasma and the supernatant extracted from myocardia were loaded onto a Sep-Pak C18 cartridge after equilibration with normal saline. The cartridge was washed with 2.5 mL normal saline and 10% acetonitrile in 0.1% trifluoroacetic acid, then eluted with 2 mL 50% acetonitrile in 0.1% trifluoroacetic acid. The elution was lyophilized and subjected to radioimmunoassay for ghrelin and GH determination. The IC50 for ghrelin was 6.95 pmol/tube and the reactivity with rat ghrelin was 100%. No cross-reactivity was seen with leptin, orexin A and B, neuropeptide Y, galanin, or vasoactive intestinal polypeptide. The lowest sensitivity of GH was 0.1 ng/mL and the reactivity with rat GH was 100%.
[3H]Ghrelin binding to myocardial sarcolemmal membrane Crude myocardial sarcolemmal membrane was prepared as previously described[18] with minor modifications, and protein content was determined by using the Bradford method[19]. The activity of membrane marker enzymes was measured as described previously by our laboratory[20]. Marker enzyme activity of membrane fractions isolated from control hearts and ISO-treated hearts showed the sarcolemmal membrane Na+-K+-ATPase activity (6.50±0.60 mmol/g protein) to be 5.8-fold higher than that of the homogenate of myocardia (0.96±0.10 mmol/g protein).
The ghrelin-receptor binding assay was carried out using [3H]ghrelin as a radioligand. The standard assay mixture contained 10 mmol/L MgCl2, 50 mmol/L Tris-HCl (pH 7.4), and [3H]ghrelin (1–40 nmol/L) in the presence or absence of unlabeled ghrelin (20 µmol/L) in a final volume of 0.2 mL. The assay mixture was preincubated at 37 ℃ for 2 min, and the binding assay was initiated by the addition of sarcolemmal membrane (50 µg protein) and allowed to proceed for 60 min at 25 ℃. At the end of incubation, the reaction mixture was diluted with 4 mL ice-cold washing buffer (10 mmol/L MgCl2, 50 mmol/L Tris-HCl, pH 7.4) and filtered immediately on Millipore membranes through 0.45-µm glass-fiber filter paper (Baxter Healthcare, Ireland) under suction. The filter paper was washed 3 times with 5 mL washing buffer (20 mmol/L Tris, 2 mmol/L MgCl2, pH 7.4) and dried, and the radioactivity was determined by using a multi-purpose scintillation counter (LS6500; Beckman, CA, USA). The specific binding was defined as the bound radioactivity displaceable by 20 µmol/L of ghrelin. All binding experiments were performed in duplicate. The maximal binding capacity (Bmax) and the affinity [the reciprocal of the dissociation constant (Kd)] for [3H]ghrelin were calculated from Scatchard plot analysis results.
Morphological analysis Hearts were isolated and the cardiac apex was fixed in 10% formalin in phosphate-buffered saline (PBS). Tissue was embedded in paraffin, and transverse sections were cut and stained with hematoxylin and eosin or 0.1% picrosirius red to stain collagen.
Statistical analysis Data are expressed as mean±SD. Comparisons between more than 2 groups were carried out by using analysis of variance (one-way ANOVA), then the Student-Newman-Keuls test. Linear regression analysis was used to assess the correlation between variables. P<0.05 was considered statistically significant.
Results
ISO induced myocardial ischemia injury and cardiac fibrosis The hearts of rats treated with ISO alone became markedly enlarged, and the heart weight and the heart coefficient (ratio of heart weight to body weight) were increased by 42% and 58%, respectively (all P<0.01) as compared with the control group. Compared with control rats, ISO-treated rats showed lower +LV dp/dtmax and -LV dp/dtmax values, decreased by 66% and 65%, respectively (P<0.01) and higher LVEDP, by 2.4-fold (P<0.01; Table 1). LDH activity in plasma was greatly increased, by 3.9-fold (P<0.01); the MDA and CD content was elevated in myocardia, by 74% and 69%, respectively (P<0.01), and in plasma, by 180% and 52%, respectively (P<0.01; Table 2). Histological sections of ISO-treated hearts showed widespread subendocardial necrosis, muscle fibers with capillary dilatation, leukocytic infiltration, swelled fibroblasts (Figure 1A), and abundant fibroblastic hyperplasia (Figure 1B).
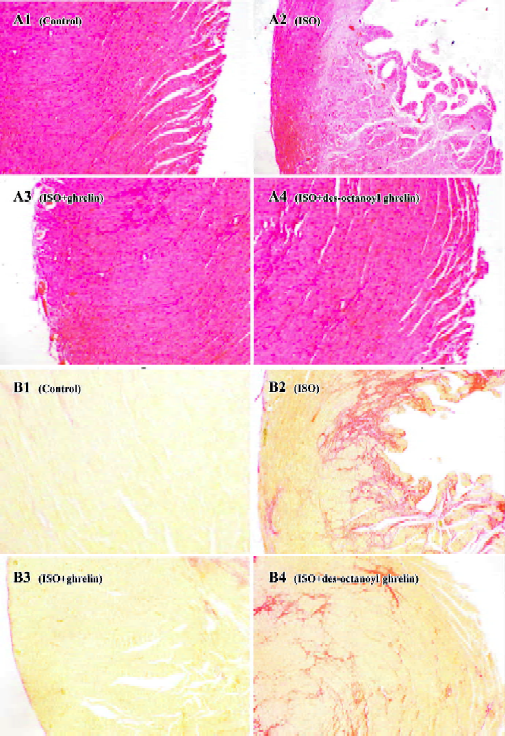
Treatment with ISO increased myocardial ghrelin content and mRNA level Compared with the control group, ISO-treated rats showed increased irradiated-ghrelin (ir-ghrelin) content in plasma by 67% (246±17 vs 410±32 nmol/L, P<0.01) and myocardia by 66% (10.41±0.57 vs 17.24+1.38 fmol/mg protein, P<0.01), and increased mRNA level in myocardia, by 93% (P<0.01; Figure 2). The ir-ghrelin content in myocardia and plasma was negatively correlated with +LV dp/dtmax (r=-0.713 and r=-0.672, respectively, both P<0.01) and -LV dp/dtmax (r=-0.668 and r=-0.584, respectively, both P<0.01); and positively correlated with LVEDP (r=0.845, P<0.01) and (r=0.864, P<0.01, respectively) but not hydroxyproline content (r=0.338 and r=0.284, respectively, both P>0.2).
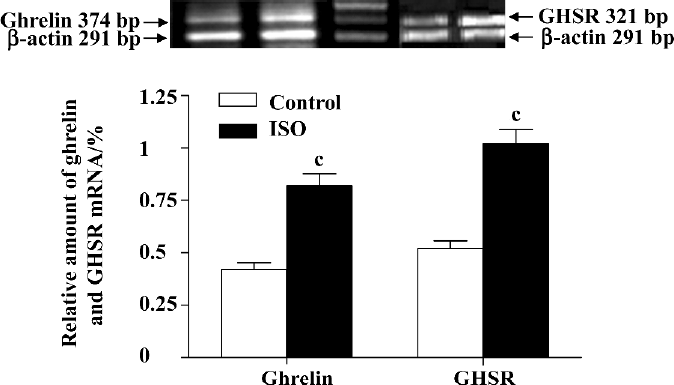
Treatment with ISO increased mRNA level of myocardial GHSR and its binding capacity Compared with the control group, ISO-treated rats showed higher GHSR mRNA levels in the myocardia, increased by 95% (P<0.01; Figure 2). [3H]Ghrelin binding to cardiac sarcolemmal membranes was found to be a saturable process, with single-component binding being characteristic of all experimental groups. In the sarcolemmal membrane, the Bmax, calculated from the results of a Scatchard plot (Figure 3), was increased by 87% in the ISO group as compared with controls (34.38±1.66 vs 18.30±1.64 fmol/mg protein, P<0.01). The affinity (Kd) for [3H]ghrelin binding in sarcolemmal membranes (Figure 3) was not significantly altered (4.31±0.42 vs 4.75±0.51 nmol/L, P>0.05).
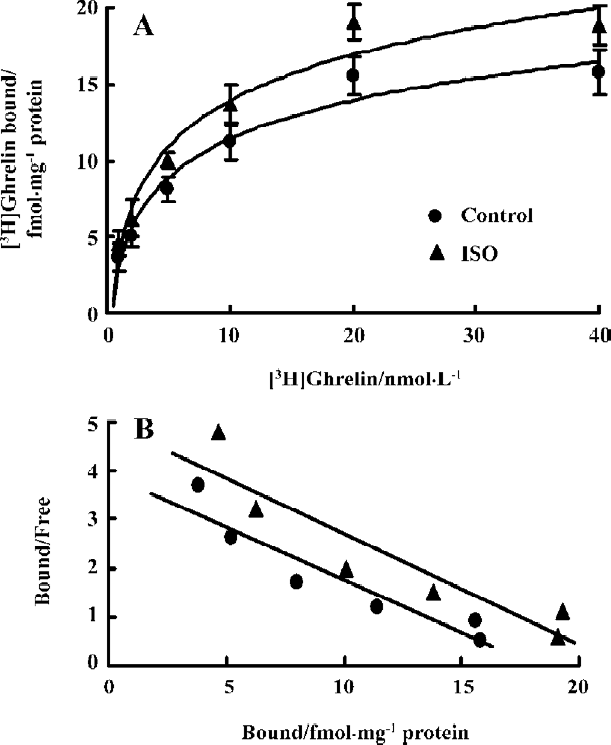
Administration of ghrelin or des-octanoyl ghrelin attenuated ISO-induced myocardial injury Compared with the ISO-alone group, rats given ghrelin showed decreased heart weight and heart coefficient by 14% and 21% (all P<0.01), respectively; +LV dp/dtmax and -LV dp/dtmax were higher, increased by 1.4- and 1.2-fold (P<0.01), respectively; and LVEDP was lower, decreased by 34% (P<0.01, Table 1). Myocyte necrosis, as indicated by myocardial LDH leakage, was ameliorated (P<0.01); and lipid peroxidation product, MDA and CD content in myocardia was obviously reduced, by 36% and 30% (P<0.01), respectively, and in plasma by 27% and 20% (P<0.01), respectively. Myocardial fibrosis, shown by hydroxyproline level, was decreased by 40% (P<0.05; Table 2). Histological sections showed that the ISO-induced myocardial injury was obviously ameliorated by ghrelin therapy, with the area stained by collagen markedly decreased (Figure 1A, 1B).
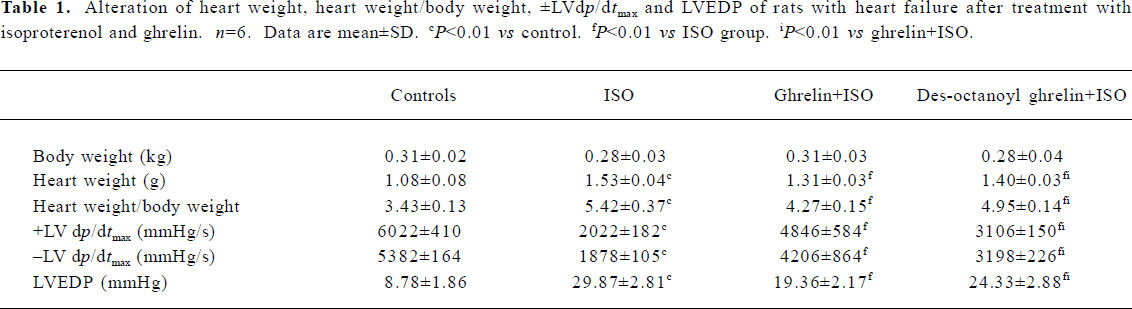
Full table
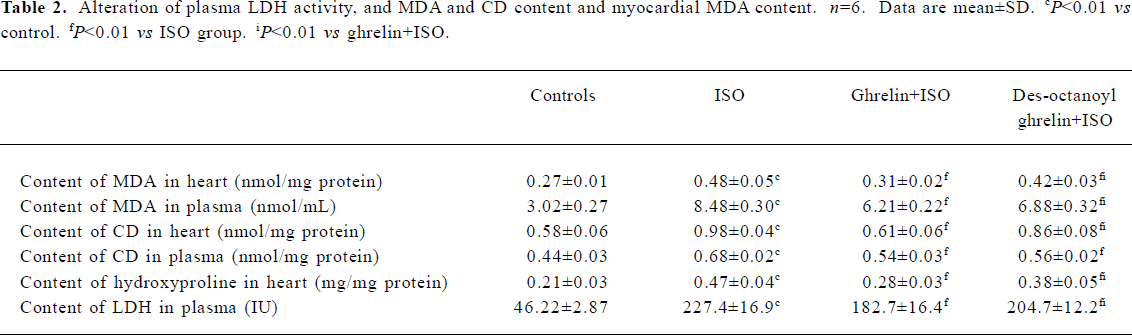
Full table
Des-octanoyl ghrelin administration also ameliorated the ISO-induced myocardial injury. Compared with the ISO-alone group, ISO-treated rats showed lower heart weight and heart coefficient, decreased by 8% and 9%, respectively (P<0.01); higher +LV dp/dtmax and -LV dp/dtmax values, increased by 54% and 70% (P<0.01), respectively, and lower LVEDP, by 19% (P<0.01; Table 1). Myocyte damage, such as myocardial LDH leakage, was ameliorated (P<0.01), and MDA and CD content in myocardia was reduced, all by 12% (P<0.01). MDA and CD level in plasma was decreased by 19% and 17% (P<0.01), respectively. Fibrosis was relieved in myo-cardia, as shown by an 18% lower hydroxyproline level (P<0.05; Table 2). Histological sections showed ameliorated ISO-induced myocardial injury and collagen deposition (Figure 1A, 1B).
The cardioprotective effects of exogenous des-octanoyl ghrelin administration on ISO-induced myocardial injury, cardiac function suppression, cardiomegaly, collagen deposition and fibrosis were markedly weaker than those of equal doses of ghrelin (P<0.05 or P<0.01; Table 2, Figure 1).
Effect of ghrelin and des-octanoyl ghrelin on plasma GH level Compared with the control group, rats treated with ISO alone showed unaltered plasma GH levels (2.88±0.31 vs 2.82±0.22 ng/mL, P>0.05); ghrelin injection greatly increased the GH level, by 1.5-fold (P<0.01), but des-octanoyl ghrelin did not affect the plasma GH level (P>0.05; Figure 4).
Discussion
Ghrelin is a strong gastrokinetic agent that links the endocrine control of energy balance and growth with the regulation of digestive function by activating its specific receptor (GHSR-1a) in the central tissues. Binding sites specific for ghrelin and its mRNA expression exist in cardiovascular tissues, which indicates that the cardiovascular system expresses the components of the ghrelin/GHSR axis, which may have an important autocrine/paracrine function in maintaining circulatory homeostasis. Intravenous injection of ghrelin has been shown to elicit dose-related decreases in arterial pressure and heart rate in conscious rabbits[21]. Treatment with ghrelin causing a direct relaxation in rat thoracic aortic rings[22] and human internal mammary arteries[23] indicated that ghrelin is a vasorelaxing factor. Enomoto and colleagues[24] showed that subcutaneous administration of 3 doses of ghrelin (1, 5 or 10 µg/kg) caused a dose-dependent increase in the LV ejection fraction in healthy volunteers, which was still apparent 60 min after ghrelin injection; in patients with chronic heart failure, an intravenous infusion of human ghrelin (0.1 mg/kg per min) significantly increased cardiac and stroke volume index[25]. These results suggest that ghrelin could be an endogenous factor with potent protective effects against cardiovascular disease.
Over-release of catecholamines is an important factor related to myocardial impairment in many cardiovascular diseases, such as myocardial ischemia, hypertrophy and heart failure. We found that treatment with high doses of ISO for 10 d resulted in severe myocardial injury, including cardio-megaly, infarction-like necrosis and massive fibrosis, as did Rona et al[13]. Cardiac function was severely inhibited, lowering the values of ±LV dp/dtmax and elevating the LVEDP. The increase in LDH activity in plasma indicated the leakage of myocardial intracellular enzymes, and the increased MDA and CD content in myocardia and plasma indicated excessive products from lipid peroxidation. The increased hydroxyproline content in myocardia and picrosirius red-stained myocardial sections indicated myocardial fibrosis. In ISO-treated rats, the mRNA levels of ghrelin and GHSR in myocardia were elevated, the [3H]ghrelin Bmax of the sarcolemmal membrane was increased, and the ir-ghrelin content in plasma and myocardia was increased, with a negative correlation between ghrelin content and cardiac function. Furthermore, exogenous administration of ghrelin effectively ameliorated the ISO-induced myocardial injury, cardiac function inhibition, cardiomegaly, collagen deposition and fibrosis. These results indicate a role for ghrelin as an endogenous cardioprotective factor.
Various agents affecting endocrine and metabolism participate in the regulation of expression, synthesis and secretion of ghrelin. Food intake, obesity and positive energy balance downregulate ghrelin level, whereas starvation and nutritional disturbance upregulate ghrelin level; insulin, growth hormone release inhibiting hormone and gastrone restrain the expression of ghrelin. However, little is known about how ghrelin and GHSR are regulated when produced and secreted in local cardiovascular tissues. In both rats and humans, the ghrelin gene, located at 3p26–p25, is made up of 4 exons and 3 introns, and the precursors contain 117 amino acids (preproghrelin). Kishimoto and colleagures[26] cloned the human ghrelin gene and characterized the 5V-flanking region, from -2000 to -1 upstream of the translation start site. The gene contained a TATATAA element and putative binding sites for several transcription factors, but not a typical GC or CAAT box. Glucagon and its second messenger cAMP enhanced the promoter activity. From our work, the upregulated myocardial ghrelin and its receptor during ISO-induced myocardial injury needs further study.
Ghrelin is a multifunctional peptide, and is crucial in regulating energy homeostasis. It induces positive energy balance by stimulating food intake, while decreasing fat utilization. In cardiovascular tissue, ghrelin has direct vaso-dilator and inotropic effects[22,23]. Ghrelin increases endothelial nitric oxide synthase (eNOS) expression and nitric oxide production[24], and interferes with endothelin-1 synthesis and release[9]. Our present work showed that treatment with ghrelin or des-octanoyl ghrelin ameliorated ISO-induced myocardial injury, and the protective effects of ghrelin were at least in part mediated by its hemodynamic potency.
It is well known that oxidative stress injury and inflammatory reactions are the main mechanisms of high-dosage ISO-induced myocardial injury. Li and colleagues[27] confirmed that ghrelin inhibited proinflammatory cytokine production, mononuclear cell binding, and nuclear factor-κB activation in human endothelial cells in vitro and endotoxin-induced cytokine production in vivo. In ISO-induced myocardial injury and in ischemia/reperfusion-induced injury in isolated rat hearts, Chang and coworkers[9] found that ghrelin effectively scavenged oxygen free radicals and inhibited lipid peroxidation injury. In our work, ghrelin and des-octanoyl ghrelin diminished leukocytic infiltration and the generation of MDA and CD, which showed that the anti-inflammatory and anti-lipid peroxidation potency of ghrelin is involved in heart protection.
Ghrelin is the only endogenous ligand of GHSR, and ghrelin-activated GHSR-1a in the pituitary gland and hypothalamus induces GH release. Many reports have found that GH administration increases cardiac performance[28], deflates infarct size and reduces unfavorable remodeling. All these effects strongly slow the invasion of heart failure[29]. Imanishi and coworkers[30] reported that GH had an anti-fibrotic effect on cardiac remodeling through a downregulation of tumor growth factor-β (TGF-β) signaling via dephosphorylation of p38 mitogen-activated protein kinase. To determine the cardioprotective mechanism of ghrelin, we compared the roles of ghrelin and des-octanoyl ghrelin in the present study. Des-octanoyl ghrelin is produced by removing octanoic acid on Ser3 from ghrelin, and lacks GH-releasing activity. Both ghrelin and des-octanoyl ghrelin effectively ameliorated ISO-induced myocardial injury, cardiac function inhibition, cardiomegaly, collagen deposition and fibrosis. The protective effect of des-octanoyl ghrelin was much weaker than that of ghrelin, which indicates that ghrelin plays its cardioprotective role in both a GH-dependent and -independent manner.
In summary, ISO-induced myocardial injury results in a compensatory increase in cardiac ghrelin and GHSR mRNA level and receptor binding capacity. Administering exogenous ghrelin and des-octanoyl ghrelin effectively attenuated ISO-induced myocardial injury, potentiated cardiac function, and ameliorated cardiac fibrosis, but the protection afforded by des-octanoyl ghrelin was markedly weaker than that of ghrelin, showing that ghrelin plays its cardioprotective role in a GH-dependent and -independent manner. Ghrelin could be an endogenous cardioprotective factor and a novel target in the prevention of and/or therapy for cardiovascular disease.
References
- Kojima M, Hosoda H, Date Y, Nakazato M, Matsuo H, Kangawa K. Ghrelin is a growth hormone-releasing acylated peptide from stomach. Nature 1999;402:656-60.
- St-Pierre DH, Wang L, Tache Y. Ghrelin: a novel player in the gut–brain regulation of growth hormone and energy balance. News Physiol Sci 2003;18:242-6.
- Shiiya T, Nakazato M, Mizuta M, Date Y, Mondal MS, Tanaka M, et al. Plasma ghrelin levels in lean and obese humans and the effect of glucose on ghrelin secretion. J Clin Endocrinol Metab 2002;87:240-4.
- Gnanapavan S, Kola B, Bustin SA, Morris DG, McGee P, Fairclough P, et al. The tissue distribution of the mRNA of ghrelin and subtypes of its receptor, GHS-R, in humans. J Clin Endocrinol Metab 2002;87:2988-91.
- Papotti M, Ghe C, Cassoni P, Catapano F, Deghenghi R, Ghigo E, et al. Growth hormone secretagogue binding sites in peripheral human tissues. J Clin Endocrinol Metab 2000;85:3803-7.
- Jeffery PL, Herington AC, Chopin LK. The potential autocrine/paracrine roles of ghrelin and its receptor in hormone-dependent cancer. Cytokine Growth Factor Rev 2003;14:113-22.
- Chang L, Ren Y, Liu X, Li WG, Yang J, Geng B, et al. Protective effects of ghrelin on ischemia/reperfusion injury in the isolated rat heart. J Cardiovasc Pharmacol 2004;43:165-70.
- Chang L, Du JB, Gao LR, Pang YZ, Tang CS. Effect of ghrelin on septic shock in rats. Acta Pharmacol Sin 2003;24:45-9.
- Chang L, Zhao J, Li GZ, Geng B, Pan CS, Qi YF, et al. Ghrelin protects myocardium from isoproterenol-induced injury in rats. Acta Pharmacol Sin 2004;25:113-7.
- Tschop M, Smiley DL, Heiman ML. Ghrelin induces adiposity in rodents. Nature 2000;407:908-13.
- Thompson NM, Gill DA, Davies R, Loveridge N, Houston PA, Robinson IC, et al. Ghrelin and des-octanoyl ghrelin promote adipogenesis directly in vivo by a mechanism independent of the type 1a growth hormone secretagogue receptor. Endocrinology 2004;145:234-42.
- Bedendi I, Alloatti G, Marcantoni A, Malan D, Catapano F, Ghe C, et al. Cardiac effects of ghrelin and its endogenous derivatives des-octanoyl ghrelin and des-Gln14-ghrelin. Eur J Pharmacol 2003;476:87-95.
- Rona G, Chappel CI, Balzas T, Gaudry R. An infarct-like myocardial lesion and other toxic manifestations produced by isoproterenol in the rat. Arch Pathol 1959;67:443-55.
- Ohkawa H, Ohishi N, Yagi K. Assay for lipid peroxides in animal tissues by thiobarbituric acid reaction. Anal Biochem 1979;95:351-8.
- Corongiu F, Banni S, Dessi M. Conjugated dienes detected in tissue lipid extracts by second derivative spectrophotometry. Free Radic Biol Med 1989;7:183-6.
- Young M, Fullerton M, Dilley R, Funder J. Mineralocorticoids, hypertension, and cardiac fibrosis. J Clin Invest 1994;93:2578-83.
- Qi YF, Bu DF, Niu DD, Shi YR, Wang SH, Pang YZ, et al. Effects of different peptide fragments derived from proadrenomedullin on gene expression of adrenomedullin gene. Peptides 2002;23:114-7.
- Wol AA, Hines DK, Karliner JS. Refined membrane preparations mask ischemic fall in myocardial beta receptor density. Am J Physiol 1989;257:H1032-6.
- Bradford MM. A rapid and sensitive method for the quantitation of microgram quantities of protein utilizing the principle of protein-dye binding. Anal Biochem 1976;72:248-54.
- Tang CS, Liu MS. Initial externalization followed by internalization of adrenergic receptors in rat heart during sepsis. Am J Physiol 1996;270:R254-63.
- Matsumura K, Tsuchihashi T, Fujii K, Abe I, Iida M. Central ghrelin modulates sympathetic activity in conscious rabbits. Hypertension 2002;40:694-9.
- Shimizu Y, Nagaya N, Teranishi Y, Imazu M, Yamamoto H, Shokawa T, et al. Ghrelin improves endothelial dysfunction through growth hormone-independent mechanisms in rats. Biochem Biophys Res Commun 2003;310:830-5.
- Wiley KE, Davenport AP. Comparison of vasodilators in human internal mammary artery: ghrelin is a potent physiological antagonist of endothelin-1. Br J Pharmacol 2002;136:1146-52.
- Enomoto M, Nagaya N, Uematsu M, Okumura H, Nakagawa E, Ono F, et al. Cardiovascular and hormonal effects of subcutaneous administration of ghrelin, a novel growth hormone-releasing peptide, in healthy humans. Clin Sci 2003;105:431-5.
- Nagaya N, Miyatake K, Uematsu M, Oya H, Shimizu W, Hosoda H, et al. Hemodynamic, renal, and hormonal effects of ghrelin infusion in patients with chronic heart failure. J Clin Endocrinol Metab 2001;86:5854-9.
- Kishimoto M, Okimura Y, Nakata H, Kudo T, Iguchi G, Takahashi Y, et al. Cloning and characterization of the 5(V)-flanking region of the human ghrelin gene. Biochem Biophys Res Commun 2003;305:186-92.
- Li WG, Gavrila D, Liu X, Wang L, Gunnlaugsson S, Stoll LL, et al. Ghrelin inhibits proinflammatory responses and nuclear factor-kappaB activation in human endothelial cells. Circulation 2004;109:2221-6.
- Volterrani M, Giustina A, Lorusso R, Giordano A. Does growth hormone play a role in chronic heart failure? Heart Fail Monit 2002;3:60-4.
- Ryoke T, Gu Y, Mao L, Hongo M, Clark RG, Peterson KL, et al. Progressive cardiac dysfunction and fibrosis in the cardiomyo-pathic hamster and effects of growth hormone and angiotensin-converting enzyme inhibition. Circulation 1999;100:1734-43.
- Imanishi R, Ashizawa N, Ohtsuru A, Seto S, Akiyama-Uchida Y, Kawano H, et al. GH suppresses TGF-beta-mediated fibrosis and retains cardiac diastolic function. Mol Cell Endocrinol 2004;218:137-46.