Baculovirus as a highly efficient expression vector in insect and mammalian cells
Introduction
Baculoviruses make up a family of viruses and are grouped into nuclear polyhedrosis viruses (NPV) and granulosis viruses. More than 500 different types of baculoviruses have been discovered and the host range is restricted to invertebrates, mostly to insects (eg, moths and butterflies); thus, several baculoviruses (eg, Helicoverpa zea, Orgya pseudotsugata, and Lymantria dispar) have been registered for use as biological pesticides by the US Environmental Protection Agency[1]. Among the numerous baculoviruses, Autographa californica multiple NPV (AcMNPV) is the most well studied and most extensively used. AcMNPV has a circular double-stranded DNA genome of approximately 130 kb, which is condensed with a protamine-like protein into the core and packed into the nucleocapsids. Nucleocapsids are synthesized in the nucleus of infected cells (typically 40 nm–50 nm in diameter and 200 nm–400 nm in length). Membrane-enveloped nucleocapsids are referred to as virus particles or virions. In nature, AcMNPV are occluded in a polyhedron (2 μm–15 μm in size) mainly consisting of polyhedrin protein. After ingestion by insects, the polyhedrin matrix is dissolved in the alkaline midgut, thus releasing the embedded virions, which subsequently infect the epithelial cells of the intestine. Early in the infection cycle, the DNA genome is replicated and transcribed in the nucleus and the nucleocapsids are assembled. The budding of nucleocapsids through plasma membrane results in the release of budded virus, which is responsible for systemic transmission within an infected insect. Late in the infection cycle, progeny nucleocapsids become membrane-bound within the nucleus and are embedded into the polyhedra. After cell death and lysis, the polyhedra are released in the wild to spread the infection. Budded virus is highly infectious to cultured insect cells and is the primary form used in the laboratory as an expression vector.
Baculovirus infection of insect cells
The baculovirus has been widely used for the production of numerous recombinant proteins in insect cells[2–4] because it has the following advantages: (i) proper post-translational modification, because insect cells are higher eukaryotes; (ii) a high capacity for multiple genes or a large insert, because of the huge and flexible viral genome (130 kb); (iii) biosafety, because baculovirus naturally does not infect humans; and (iv) a very high yield driven by the strong promoters polyhedrin or p10. Among the natural host cells, Sf-9 and Sf-21, derived from the ovarian tissue of Spodoptera frugiperda, and BTI-TN-5B1-4 (High-Five; Invitrogen, Carlsbad, CA, USA), derived from Trichoplusia ni, which is suitable for the expression of secreted proteins[5], are most popular. In addition to cultured cell lines, insect larvae are also excellent hosts for protein production. For example, T ni insect larvae can be readily infected by recombinant AcMNPV[6,7], and silkworms can be readily infected by recombinant Bombyx mori NPV[8]. Both systems have been employed for the production of numerous recombinant proteins[6,9]. The products synthesized in insect larvae are almost identical to those produced in insect cells and mammalian cells and the yields are generally very high (eg 13 mg luciferase per larva[10]). Therefore, baculovirus infection of live insects may represent an inexpensive and rapid approach for protein production[11], but consistent infection and qua-lity control are more difficult to achieve.
The large cloning capacity renders baculovirus an ideal tool for the synthesis of virus-like particles (VLP), which generally require simultaneous expression of multiple viral structural proteins for self-assembly. VLP are empty particles composed of viral structural proteins but devoid of viral nucleic acids; thus, they are non-infectious. VLP can generally induce broad and strong immune responses thanks to the preservation of many essential epitopes[12,13]; therefore, VLP have gained increasing attention as potential vaccine candidates[14,15] or diagnostic reagents[16]. Extended applications of VLP as gene delivery vehicles have also been reported[17,18]. To date, numerous VLP of different viruses, including HIV[19], herpes simplex virus[20], human papilloma-virus[21], polyomavirus[22], parvovirus[23], infectious bursal disease virus[24], hepatitis C virus (HCV)[16], and enterovirus 71[25], have been expressed using the baculovirus/insect cell expression system. Very recently, the synthesis of severe acute respiratory syndrome coronavirus (SARS-CoV) VLP using the baculovirus/insect cell system has also been reported[26,27]. The assembly of VLP using baculovirus enables the investigation of virus assembly process as well as the minimal requirements for viral capsid assembly. For example, Mortola and Roy demonstrated that coexpression of SARS-CoV envelope and membrane proteins is required and sufficient for the assembly of spikeless VLP[27], yet additional coexpression of the spike (S) protein results in the incorporation of the S protein. The resultant VLP possesses distinctive spike projections, making it morphologically indistinguishable from the authentic virus. Through co-infection with different recombinant baculoviruses, one can manipulate the composition of the particles, obtaining higher specific immunogenicity[28], or other biological functions by incorporating enzymes[29] or affinity tags for downstream purification[24]. In this regard, Conner et al successfully demonstrated chimeric VLP formed by structural proteins of viruses isolated from different animals (bovine and simian)[30]. By including viral proteins from different strains, referred to as phenotypic mixing, one can broaden the spectrum of the chimeric particles as a vaccine[31].
There are drawbacks associated with the baculovirus/insect cell expression system. First, baculovirus infection ultimately results in cell death and lysis in a few days. Because the expression driven by a polyhedrin or p10 promoter usually reaches the maximum near the death of infected cells, protein processing is likely to be suboptimal at that time because of the compromise of post-translational machinery and secretory pathway. This may seriously affect the processing of proteins destined for plasma membrane or for secretion. Efforts have been directed towards alleviating this problem by the use of early baculovirus promoters (eg, IE1) in either transiently or stably transformed cells[32]. The expression driven by early promoters is continuous and stable, and confers more efficient processing of the glycoprotein (eg tissue plasminogen activator), but the expression level is lower than those obtained with the lytic baculovirus system. Such a system is a feasible approach for expression in insect cells for products that are relatively poorly processed in lytic insect cells, and may be useful for functional studies of recombinant receptor proteins. To circumvent the protein degradation problem associated with cell lysis, a novel non-lytic baculovirus has recently been developed by random mutagenesis of viral genomes[33] and subsequent selection. At 5 d post-infection, the non-lytic baculovirus shows only 7% cell lysis, in contrast to 60% cell lysis by lytic baculovirus. This system is thus a convenient alternative for the production of proteins vulnerable to degradation using lytic baculoviruses.
The second disadvantage is that glycosylation in insect cells differs in many aspects from in mammalian cells. For example, truncated oligosaccharides containing just 3 or even 2 mannose residues and sometimes fucose have been found on proteins expressed in insect cells. Besides, there appears to be no significant sialylation of N-glycans in insect cells[34]. The glycoproteins lacking sialic acids have extremely short half-lives in vivo; therefore, the truncated N-glycans of glycoproteins produced in insect cells constitute a barrier to their use as therapeutics. One example to engineer the N-glycosylation pathway is the construction of transformed Sf-9 cells that constitutively express bovine β1, 4-galacto-syltransferase under hr5-ie1 control[35,36]. Baculo-virus infection of this cell line results in the production of terminally galactosylated recombinant proteins. Other attempts and strategies to “mammalianize” or “humanize” the N-glycosylation capacity of insect cells have been reviewed previously[37–39]. Another limitation of the baculovirus/insect cell system is its inefficiency to properly process proteins that are initially synthesized as large inactive precursor proteins, such as peptide hormones, matrix metalloproteases, and fusogenic viral envelope glycopro-teins. A family of mammalian proprotein convertases has been characterized to be involved in the cleavage, and it has been shown that coexpression of the prohormone convertase furin with transforming growth factor β1 (TGF β1) results in a 7.8-fold increase in the production of mature TGF β1[40].
Baculovirus transduction of mammalian cells
Baculovirus was originally regarded as infecting solely insects and invertebrates; but in 1983 Volkman and Goldsmith found that baculovirus can be internalized by non-target vertebrate cells (eg, human lung carcinoma cell line A427)[41]. Carbonell et al further confirmed the entry of baculovirus into mammalian cells and very low level reporter gene expression under the control of polyhedrin and Rous sarcoma virus (RSV) promoters in mammalian cells[42]. However, virus replication was not detected in either study. Their discovery was not widely noted until 10 years later when two pioneer groups reported that recombinant baculoviruses harboring a cytomegalovirus (CMV) promoter-luciferase gene cassette[43] or an RSV long terminal repeat (LTR) promoter-β galactosidase (β-gal) gene cassette[44] can efficiently transduce mammalian cells. Their data indicate a strong preference for baculovirus to enter hepatocytes of different origins, and suggest that the block to expression in less susceptible cells does not appear to result from the ability to be internalized by the target cells but rather by events subsequent to viral entry because high and low expressing cell lines internalize similar amounts of virus[44]. One factor accounting for the low apparent transduction efficiency in other cell types is promoter strength. Shoji et al showed that cells that are not transduced by a baculovirus expressing β-gal under the control of a CMV promoter can be efficiently transduced by a baculovirus expressing the same reporter protein under the transcriptional control of a stronger CAG promoter[45] (a composite promoter consisting of the CMV immediate early enhancer, chicken β-actin promoter and rabbit β-globin polyadenylation signal). Thus, it is of interest to examine different promoters of viral and cellular origins in the baculovirus context in mammalian cells. Following these findings, subsequent studies have rapidly expanded the list of permissive cells that include cell lines originating from human (eg, HeLa, Huh-7, HepG2, keratinocytes, and bone marrow fibroblasts), rodent (eg, CHO, BHK), porcine (eg, CPK, PK-15), bovine (eg, BT)[46], and even fish sources (eg, EPC, CHH-1)[47]. Besides, baculovirus is capable of transducing non-dividing cells[48]. Transduction of primary cells, such as human neural cells[49], pancreatic islet cells[50], and rat articular chondrocytes[51], has also been observed. In addition, Wagle and Jesuthasan recently showed that baculovirus can successfully transduce the embryos of zebrafish[52]. By injecting the baculovirus expressing ephrinB2a into specific tissues, ephrinB2a is normally expressed in the posterior region of developing somite and baculovirus-mediated misexpression causes abnormal somite boundary formation. Despite the rapidly growing list of susceptible cells, however, baculovirus transduction of cell lines of hematopoietic origin, such as U937, K562, Raw264.7[53], LCL-cm, and Raji[54], is inefficient.
The transduction efficiencies vary considerably depending on cell types and can be up to 95% for BHK cells or lower than 10% for NIH-3T3 cells[54]. One possibility accounting for the high transduction efficiency in certain cell lines is the activation of mammalian promoters (eg, CMV promoter and heat shock promoter) by a DNA sequence upstream from the polyhedrin (pu) and a homologous region (hr) of AcMNPV[55]. The hr1 sequence enhances transcription from polyhedrin and Drosophila heat shock protein (hsp70) promoter in Sf cells[56] and functions as an origin of replication (ori), but does not support ori activities in mammalian cells[57]. pu and hr together function synergistically, resulting in as much as 18 000-fold promoter activation[55]. The hr1 can also function in mammalian cells as an enhancer when present in trans[57]. The insertion of an additional copy of the hr1 region in the AcMNPV genome thus represents an attractive approach to promoting overexpression of foreign proteins in mammalian cells as has been demonstrated in insect cells[56]. The additional hr1 can also help maintain the genetic stability of the bacmid-derived baculoviruses, because spontaneous deletion of the heterologous gene(s) in the foreign bacterial artificial chromosome sequences readily occurs[58,59].
The transduction efficiency can be markedly enhanced by the addition of sodium butyrate, trichostatin A[53], and valproic acid[60]. These compounds are histone deacetylase inhibitors, inducing a hyperacetylation of the chromatin and enhancement of transcription, thus highlighting the importance of the chromatin state of the baculovirus genome in the transduced cells for transgene expression. However, cytotoxicity is often associated with the use of these drugs[60]. Another approach to enhance the transduction efficiency is to alter the transduction protocol. For transduction, typically the virus is concentrated by ultracentrifugation and resuspended in phosphate-buffered saline (PBS). The cells are then incubated with the virus for 1 h at 37 °C using growth medium (eg, DMEM) as the surrounding solution[44,45,61]. Recently, we found that incubation of the unconcentrated virus (ie virus supernatant) at a lower temperature (eg, 25 °C) for 4 h–6 h using PBS as the surrounding solution results in gene transfer into HeLa and chondrocytes[51,62] with efficiencies comparable or superior to those using the traditional protocols. This is in part because of prolonged virus uptake provided that sufficient amount of the virus is present. One key determinant accounting for the high transduction efficiency is PBS, which is superior to DMEM or TNM-FH (the medium for baculovirus production) as the surrounding solution in terms of enhancing the transduction efficiency and transgene expression[51,62], although the exact mechanism awaits further elucidation. The same protocol also confers gene delivery into human mesenchymal stem cells (MSC) isolated from umbilical cord blood (uMSC) and bone marrow (bMSC) with efficiencies of up to approximately 72.8% and 41.1%, respectively[63] (Figure 1). This protocol eliminates the need for virus ultracentrifugation; thus, it not only represents a simpler approach, but also considerably reduces possible virus inactivation during ultracentrifugation.
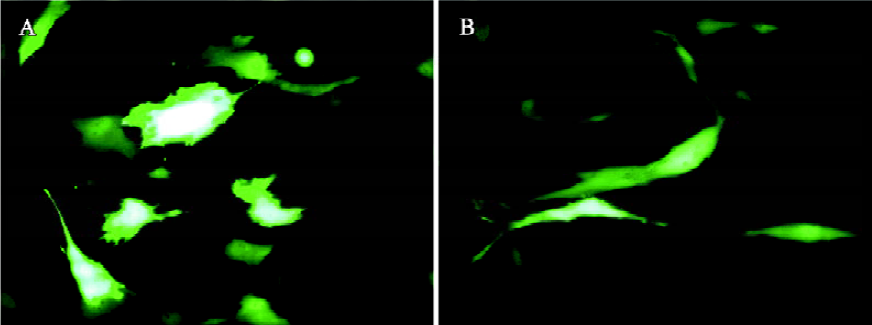
Baculovirus transduction is generally considered non-toxic to mammalian cells and does not hinder cell growth even at high multiplicity of infection[43,64]. Our recent studies again confirm this notion because transduction with a wild-type baculovirus does not cause any observable adverse effect to chondrocytes or MSC[51,63]. From the growth curves, however, we noted a slower cell proliferation on expression of enhanced green fluorescent protein (EGFP) under the transcriptional control of a CMV promoter. The slightly retarded cell growth is attributed to the EGFP overexpression because: (i) EGFP overexpression could be toxic and might even induce apoptosis in some cells[65,66]; and (ii) the transcriptional activation of CMV promoter could repress other viral or cellular gene expression[67]. Fortunately, the cell growth rate may be restored after several passages, as EGFP expression attenuates[63].
Mechanisms of baculovirus entry into mammalian cells Baculovirus enters insect cells via endocytosis followed by low pH-induced fusion of a viral envelope protein with the endosomal membrane, thus allowing viral entry into the cytoplasm and nucleus[68]. Likewise, baculovirus is generally considered to follow the same route to enter mammalian cells, as gene expression is inhibited by lysosomotropic agents (eg, chloroquine), which block endosomal matura-tion[43,44]. Initially it was suggested that the transduction is liver specific and the asialoglycoprotein could be involved in virus binding[43,44]. However, van Loo et al showed that Pk1 cells, which do not express the asialoglycoprotein rece-ptors, could be successfully transduced, thus asialoglyco-protein is not a key determinant[48]. It was also shown that electrostatic interactions and the heparan sulfate moieties might be necessary for baculovirus binding to the mammalian cell surface[69]. However, no follow-up report has confirmed their findings and the nature of the receptors remains unclear. In contrast, the viral envelope glycoprotein gp64 is shown to be essential for virus attachment and endosomal escape[70]. Supporting this is the finding that a baculovirus over-expressing gp64 in addition to the endogenous copy of gp64 can incorporate approximately 1.5-fold the normal amount of gp64 on the virion surface and exhibit 10- to 100-fold more reporter gene expression in a variety of mammalian cells compared with similar viruses with a normal amount of gp64[71]. In the same study, phospholipids on the cell surface are suggested to serve as an important docking point for gp64, thus facilitating viral entry into mammalian cells. By transient depletion of calcium using EGTA pretreatment, Bilello et al demonstrated that paracellular junction complexes are important for baculoviral entry into primary hepatocytes[72].
It is generally assumed that the escape from the endo-somes sets the block for transduction of some mammalian cells by baculovirus[44,73]; however, a recent study showed that the transduction block lies not in the endosomal escape, but rather in the cytoplasmic trafficking or nuclear import of the virus capsids[74]. In the cytoplasm, the nucleocapsids appear to induce formation of actin filaments, which probably facilitate the transport of viral nucleocapsids into the nucleus, as cytochalasin D strongly reduces the transduction efficiency but not the delivery of nucleocapsids to the cytoplasm[48]. In contrast, we recently found that incubation of cells with PBS during transduction results in significantly higher virus entry and higher transduction efficiency compared with incubation with DMEM[63], suggesting that virus internalization may be a bottleneck as well. Overall, the events responsible for virus uptake and detailed mechanisms of intracellular movement and nuclear entry of the virus are still largely unknown.
Baculovirus display The tropism and transduction efficiency of baculovirus has been manipulated by modifying the envelope protein. The modification can be performed by fusing a heterologous protein (or peptide) in-frame at the N-terminus of the gp64 gene under the control of a polyhedrin or p10 promoter. The fusion protein, after expression as an additional copy, is translocated to the plasma membrane and incorporated into the viral envelope on virus budding. Proof of this principle was first approached by fusing HIV-1 envelope proteins; the modified virus binds the CD4 receptor on T cells[75]. A similar strategy has been applied to construct avidin-displaying baculovirus, which shows a 5-fold increase in transduction efficiency in rat malignant glioma cells and a 26-fold increase in rabbit aortic smooth muscle cells compared with the wild-type baculovirus[76]. Baculovirus displaying heterologous envelope proteins, such as vesicular stomatitis virus G protein (VSVG), has also been constructed, which transduces human hepatoma and rat neuronal cells at efficiencies roughly 10- to 100-fold greater than baculovirus lacking VSVG[73]. This pseudotyped virus also transduces cell lines that are transduced at very low levels or not at all by the unmodified baculovirus, thus broadening the tropism. The enhanced transduction efficiency and wider tropism are attributed to increasing transport of baculovirus DNA into nuclei rather than to increasing cell binding or virus uptake[73]. In contrast, specific targeting of baculovirus to mammalian cells by displaying a single-chain antibody fragment specific for the carcinoembryonic antigen (CEA) or synthetic IgG binding domains is also demonstrated[77,78]. In addition, the baculovirus display technology has been utilized to construct and screen a eukaryotic epitope library[79]. In this context, the HIV-gp41 epitope “ELDKWA” specific for the neutralizing MAb 2F5 is inserted into the antigenic site of influenza virus hemagglutinin, flanked by additional random amino acids. This pool of hemagglutinin genes is cloned into baculovirus, and an individual clone displaying the epitope with markedly increased binding capacity out of a pool of 8000 variants is screened rapidly by fluorescence activated cell sorting.
Note, however, that the expression of gp64-fusion under the control of a late promoter may lead to a heterogeneous virus population that possesses various amounts of fusion protein and hence varied properties. This might be resolved by: (i) inserting the gp64 fusion gene into the genomic gp64 locus so that all gp64 copies contain the fusion partner; and (ii) expressing the gp64 fusion protein under an early promoter. More recently, baculovirus capsid display has also been developed in which the fusion can be made to the N- or C-terminus of the major capsid protein vp39 rather than to gp64 without compromising the viral titer or functionality[74]. It has been demonstrated that the incorporation of green fluorescent protein (GFP) into the virus capsid does not interfere with the capsid assembly and allows for visualization of the virus biodistribution in vivo[74]. This capsid-modified baculovirus holds great promise for the nuclear and subcellular targeting of the transgenes.
Viral vector generation and viral protein production Thanks to the large DNA genome, recombinant baculovirus offers an attractive means for the production of other viral vectors for gene delivery/gene therapy purposes because the production of many viral vectors requires either plasmid transfection into producer cell lines or helper virus infection. For instance, all genes essential for replication and packaging of the adenoviral vector (38 kb) can be delivered and expressed by a single baculovirus, which is co-transfected with a plasmid vector harboring only the inverted terminal repeats (ITR) and packing signal of the wild-type virus as well as a reporter gene[80]. The co-delivery results in the generation of a fully deleted adenovirus vector free of helper virus. This technique is also exploited for the production of adeno-associated virus (AAV), whose use is hampered by the lack of a simple and efficient vector production method. The mammalian producer cells are co-transduced with 3 viruses: 1 baculovirus harboring the reporter gene flanked by AAV ITR, 1 baculovirus expressing the AAV rep gene, and a helper adenovirus expressing the AAV cap gene[81]. The simultaneous delivery produces infectious recombinant AAV particles and constitutes an option for improving large-scale recombinant AAV vector production. Besides virus preparation, Ramos et al recently investigated the feasibility of using the baculovirus/mammalian cell system for protein production. They investigated two protocols for optimal cell culture and virus transduction and achieved volumetric yields for 3 secreted proteins (SAF-3-Fc, CD40-hexa his, and Asp 2-Fc) at 4 mg/L–25 mg/L[82]. In our laboratory, the application of baculovirus is further expanded to the production of hepatitis delta virus (HDV) VLP. HDV can not replicate in cultured cell lines, and thus is difficult to propagate; therefore, studies of HDV particle assembly mainly rely on cDNA transfection, which suffers, however, from low efficiency and hinders subsequent structural and immunological studies of HDV VLP. We demonstrated that the co-transduction of hepatoma cell lines with 2 recombinant baculoviruses, one expressing hepatitis large delta antigen and another expressing hepatitis B surface antigen (HBsAg), leads to self-assembly and secretion of the HDV VLP[83]. The yield (~150 ng HBsAg per mL in the secreted particle) is approximately 2-fold of that achieved by plasmid transfection. VLP synthesis has also been demonstrated in BHK cells and the process is transferred to a novel oscillating bioreactor for production on a larger scale[84]. One bioreactor run (500 mL working volume) can yield over 400 μg HBsAg, which substantially increases the total particle yield and thus enables future structural and immunological studies of the VLP. In comparison with stable cell lines, the gene expression levels and the VLP composition can be easily manipulated simply by varying the virus concentration. In addition, the expressed proteins are obtained in a few days; therefore, this baculovirus/mammalian cell expression system may serve as a transient expression system for early evaluation of proteins of interest while stable cell lines are being generated and evaluated.
Studies of gene functions and viral infections Gene delivery into primary cells using transfection or electroporation either suffers from low efficiency or causes extensive membrane damage. Because baculovirus is generally non-toxic and non-replicative in mammalian cells, and the transduction efficiencies into various human primary cells are high, cell-based assays have been developed for gene function studies. Clay et al demonstrated that co-transduction of cells with 2 recombinant baculoviruses, one expressing an estrogen receptor and another expressing a reporter gene controlled by an estrogen receptor-responsive promoter, results in ligand-induced reporter gene activity, and this approach is successfully applied to assay development in Saos-2 human osteosarcoma (HOS) cells[85]. Similarly, a cell/cell fusion assay that mimics the HIV viral/cell fusion process was developed based on the baculovirus system[86]. In the assay, HOS cells stably expressing CCR5, CD4, and LTR-luciferase serve as the recipient host cell. An HEK-293 cell line transduced with a baculovirus expressing the viral proteins gp120, gp41, tat, and rev represents the virus. Interaction of gp120 with CCR5/CD4 results in the fusion of 2 cells and transfer of tat to the cytoplasm of the HOS cell; tat, in turn, binds to the LTR region on the luciferase reporter and activates transcription, leading to an increase in cellular luciferase activity. This assay has been demonstrated to be a robust and reproducible high-throughput surrogate assay for evaluating the effects of compounds on gp120/CCR5/CD4-mediated viral fusion into host cells[86]. This system could also facilitate the assessment of different promoters for their strength as well as temporal and spatial regulation across mammalian cells of different origins.
Similar to HDV, hepatitis B virus (HBV) and HCV are not capable of replication in cultured cells; thus, the evaluation of novel antiviral strategies have been hindered by the lack of a convenient and reliable in vitro culture system. To overcome this problem, recombinant baculoviruses are constructed to carry and deliver the genomes of HBV[87] and HCV[88] into hepatoma cells lines. With this approach, replication of HBV DNA and RNA, and expression of viral genes are detected[87]. Viral replication is evidenced by the presence of high levels of intracellular replicative intermediates and protected HBV DNA in the medium. The successful HBV replication thus allows for the establishment of a highly flexible system for studying: (i) the effects of (-)-β-2',3'-dideoxy-3'-thiacytidine on HBV replication and accumulation of covalently closed circular DNA[89]; and (ii) the cross-resistance profiles of HBV mutants against antihepadnaviral compounds[90]. The HCV full-length and minigenome under the tetracycline-inducible promoter are also delivered into HepG2 cells and regulable transcription and viral polypeptide processing are demonstrated[91], thus providing a novel tool for the analysis of HCV replication and host-cell interactions.
Generation of stable cell lines Normally, baculovirus-mediated gene expression lasts approximately 1–2 weeks as a result of cell division. The gradual extinction of transgene expression is also attributed to the degradation of baculoviral DNA, as degradation has been revealed in hepatoma cells[83] and articular chondrocytes[51]. However, it was found that transduction of CHO cells with a baculovirus expressing neomycin phosphotransferase results in the isolation of CHO cells stably expressing GFP by selection using the antibiotic G418[53]. The frequency of G418 resistant colony formation is approximately 1 in 50–100 of the transduced cells. The same group further confirmed that discrete portions of baculovirus DNA are randomly integrated as single-copy fragments ranging in size from 5 kb to 18 kb[92]. Periodic assays on the stable clones show no loss of transgene expression over a 5 month period. Coupled with high transduction efficiency, the high frequency of emergence of stable transductants makes baculovirus a valuable tool to derive human cell lines that are either difficult to isolate in large numbers or are difficult to transfect by other methods.
In vivo gene therapy Thanks to highly efficient gene delivery, baculovirus has captured increasing interest as a vector for in vitro and in vivo gene delivery. However, reports of successful in vivo applications are limited. The complement system appears to be a potent barrier for in vivo gene therapy because the efficiency of gene transfer into hepatocytes of complement-deficient mice is greatly enhanced[70]. Inactivation of baculovirus in human plasma and whole blood is prevented by treatment with cobra venom factor[93]. An alternative approach is adopted by avoiding contact of baculovirus vectors with blood components. By using a silastic collar, transduction of adventitial cells in rabbit carotid arteries is achieved and the efficiencies are comparable to those obtained with adenoviral vectors[94]. The gene expression is transient and the arterial structure and endothelium remain intact after baculovirus transduc-tion, although signs of inflammation are detected. Unmodified baculovirus vectors have also been injected into the rodent brain where the complement proteins may not reach in the presence of an intact blood-brain barrier and/or the complement level in the brain is insufficient to affect the gene transfer[95]. After in vivo injection into the brain, baculovirus specifically transduces the epithelium of the choroids plexus in ventricles and the transduction efficiency is as high as 76%±14%; thus, baculovirus seems to be especially useful for the targeting of choroids plexus cells[95].
A more cutting-edge approach to alleviate the complement inactivation problem is the generation of complement-resistant baculovirus by displaying decay-accelerating factor, a regulator that blocks complement at the central step of both the classical and alternative pathways[96]. Such a complement-resistant baculovirus vector expressing additional human coagulation factor IX (hFIX) allows for a substantial improvement of gene transfer into complement-sufficient neonatal rats in vivo after local injection into the liver parenchyma. Gene expression is transient probably as a result of the generation of antibodies directed against the transgene product hFIX, which might lead to clearance of either expressed hFIX protein and/or positively transduced cells. Alternatively, baculovirus can be pseudotyped by displaying VSVG on the envelope. The VSVG-modified virus enhances gene transfer efficiencies into mouse skeletal muscle in vivo and the transgene expression lasts 178 d in DBA/2J mice and 35 d in BALB/c and C57BL/6 mice[97]. The VSVG-modified baculovirus also exhibits greater resistance to inactivation by animal sera and can transduce the cerebral cortex and testis of mice by direct inoculation in vivo[61].
Another potential barrier may be the presence of heparin or heparin-like factors, which block baculovirus-mediated gene delivery in cell culture[93]. Intercellular junctions may be an additional hurdle because transient disruption of these junctions by EDTA treatment prior to transduction improves the gene delivery efficiency into long-term cultures of primary hepatocytes but does not lead to permanent alternations in hepatocyte ultrastructure, albumin mRNA, and protein expression profiles[98]. Their studies also suggest the importance of the basolateral surface for virus entry at least for some cell types[72]. In our laboratory, however, the transient disruption of cell junctions fails to effectively enhance the baculovirus-mediated gene transfer into chondrocytes and HepG2 cells that are cultured to over-confluence, implying that other factors in addition to the paracellular junction complexes might be involved in the transduction of these cells.
Another problem associated with baculovirus as a gene delivery vector is that purification by ultracentrifugation often leads to virus aggregation[99]. In addition, the ultracentrifugation process is time-consuming, labor intensive, and difficult to scale up. To resolve these problems, we construct a recombinant baculovirus with a hexahistidine (His6) tag displayed on the viral envelope, which enables virus purification by a simple immobilized metal affinity chromatography with high purity (~87%). This methodology obviates the need for successive ultracentrifugation steps[100] and may be employed to purify other viral vectors for gene therapy studies.
Ex vivo gene therapy To date, most gene therapy studies using baculovirus vectors have focused on in vivo experiments, yet relatively little is known about the potential of baculovirus for ex vivo therapy. One relevant report was published by Sandig et al[101] who established an ex vivo perfusion model for human liver segments. The recombinant baculovirus is perfused through the liver segments for 15 min and reasonable transduction rates are achieved in all perfused parts of the liver tissue. This study verifies for the first time that baculovirus-mediated gene transfer is possible in the liver tissue and encourages future studies including in situ perfusion of intact livers with baculovirus vectors in animal models.
Recently, we have also demonstrated the highly efficient baculovirus-mediated gene transfer into articular chondro-cytes[51] and human MSC[63], both being candidate cell sources for cartilage tissue engineering. Importantly, normal differentiation states of chondrocytes and MSC are retained on baculovirus transduction. Our experiments regarding MSC suggest that the phenotypic changes along the adipogenic differentiation pathway positively influence the baculovirus transduction and may implicate two possible applications: (i) MSC may be transduced with baculovirus expressing specific factors (eg b-FGF or BMP-2) to promote the expansion or regulate the differentiation via autocrine and paracrine effects of the factors so that supplementation of these factors in the medium may be omitted; and (ii) differentiation of MSC towards a specific lineage pathway may be induced first and the committed progenitor cells can be transduced ex vivo by the recombinant baculovirus expressing appropriate growth factors (eg TGFβ1 or IGF-1), seeded onto polymeric scaffolds, and implanted into animal models for tissue regeneration. Although sustained transgene expression is restricted by the degradation of baculoviral DNA within the cells, the non-replication and degradation characteristics prove that baculovirus may be a safe gene delivery vehicle for tissue engineering in which long-term expression is not critical.
Immunological response and potential as a vaccine vector Host responses to baculovirus uptake, either in vitro or in vivo, were not evaluated until Gronowski et al reported that administration of baculovirus in vitro induces interferon (IFN) production from human and murine cell lines and induces in vivo protection of mice from encephalomyocarditis virus infection[102]. A more recent study discovered that the presence of baculovirus disrupts the phenobarbital gene induction, a potent transcriptional activation event characteristic of highly differentiated hepatocytes, and represses expression of the albumin gene, but neither cAMP nor PKA activities are affected by the virus[102]. Baculovirus transduction also induces expression of cytokines such as TNF-α, IL-1α, and IL-1β in primary hepatocytes[103]. These findings raise concerns as to whether these properties will compromise the use of baculovirus vectors for in vivo gene therapy in humans, and more investigations will be needed to ensure the safety of baculovirus vectors. Despite these, the ability of baculovirus to induce immune responses has been exploited by Abe et al, who found that intranasal inoculation with a wild-type baculovirus elicits a strong innate immune response that protects mice from a lethal challenge of influenza virus[104]. The feasibility of using baculovirus as a vaccine carrier was also demonstrated by Aoki et al[105], who found that a recombinant baculovirus expressing glycoprotein gB of pseudorabies virus induces antibodies against gB protein in mice, thus suggesting that this recombinant baculovirus could serve as a vaccine candidate for pseudo-rabies. Similarly, in vivo administration of a baculo-virus expressing hFIX elicits antibodies against hFIX. The antibody titers are proportional to the amount of protein produced by the virus[96]. More recently, Facciabene et al demonstrated that the intramuscular injection of a baculovirus expressing CEA induces measurable anti-CEA-specific CD4+ T-cell response[106]. The immunogenic property of baculo-virus is not restricted to CEA because the intramuscular injection of another baculovirus (Bac-E2) expressing E2 glycoprotein of HCV induces anti-E2 CD8+ T-cell response[106]. Interestingly, when Bac-E2 is pseudotyped to display VSVG on the envelope, the minimal dose required to elicit a measurable T-cell response is 10-fold less, indicating that the VSVG-pseudotyped Bac-E2 appears to be a more potent immunogen than the unmodified virus. This finding agrees with the previous statement that baculovirus displaying VSVG confers a more efficient immunogen expression in transduced cells.
It is also likely that baculovirus can mediate an immune response against an antigen when it is displayed on the viral surface. In this regard, the immunodominant antigenic site of foot-and-mouth disease virus (FMDV) has been displayed on the baculovirus envelope[107], which is able to bind a panel of FMDV-specific MAb and indicates the preservation of antigenic epitopes on the virus envelope. A more recent study further showed that immunization with adjuvant-free baculovirus displaying rodent malaria Plasmodium berghei circumsporozoite protein (PbCSP) on the envelope induces high levels of antibodies and γ-interferon-secreting cells against PbCSP, and protects 60% of mice against sporozoite challenge[108]. These studies substantiate the potential of baculovirus displaying immunogens as a vaccine candidate.
Conclusions and future prospects
The values of baculovirus for recombinant protein expression in insect cells have long been proven. The extremely high yield by baculovirus-infected insect cells or larvae makes it an attractive tool for pharmaceutical protein production. Despite its inability to process some proteins correctly, rapid advances in cell engineering may overcome these drawbacks and render baculovirus a proper system for large-scale protein production. To the best of my knowledge, no insect cell-derived recombinant protein has gained official approval from the US Food and Drug Administration. Nonetheless, more and more proteins are produced in insect cells in the preclinical stage. Particularly promising is the field of viral vaccines, where the formation of virus-mimicking multimers can be essential for eliciting protective immune responses. An HCV vaccine might be the first human therapeutic expressed in insect cells[109].
The broad range of susceptible mammalian cells, coupled with its non-toxic and non-replication nature, makes baculovirus a useful tool for studying the expression and function of gene products. As a gene therapy vector, baculovirus possesses the following extra advantages: (i) the AcMNPV genome is large, thus rendering the virus flexible to carry multiple genes or large inserts; (ii) recombinant baculoviruses are easy to construct and produce to high titers simply by infecting insect cells; and (iii) the purification of baculovirus can be readily performed by ultracentrifugation[110] or, alternatively, by a newly developed affinity chromatographic technique[100]. The last two features greatly simplify the large-scale preparation of high titer baculoviral vectors. In addition to therapeutic genes, baculo-virus may serve as a promising vector for the delivery of cDNA of infectious RNA viruses towards antisense therapy approaches. The same concept may be applied to the delivery of siRNA to silence specific target genes. The combination of ex vivo gene transfer and tissue engineering holds great promise as well. One bottleneck to the application of baculovirus lies in the short duration of transgene expres-sion. This may be overcome by selecting a proper promoter that is not silenced, and developing a proper superinfection protocol that can effectively deliver fresh genes into cells. The baculovirus may also be applied in conjunction with other viral vectors to escape either pre-existing or therapy-induced antiviral immunity. In summary, baculovirus can be used as an efficient vector for gene delivery into insect and mammalian cells for a wide variety of applications.
Acknowledgment
The author gratefully acknowledges the financial support of the National Science Council (Grant NSC 93-2214-E-007-016) and Ministry of Economic Affairs (Technology Development Program for Academia Grant 93-EC-17A-17S1-0009), Taiwan, China.
References
- Wood HA. Development and testing of genetically improved baculovirus insecticides. In: Shuler ML, Wood HA, Granados RR, Hammer DA, editors. Baculovirus expression systems and biopesticides. New York: Wiley-Liss; 1995. p91–102.
- Patterson RM, Selkirk JK, Merrick BA. Baculovirus and insect cell gene expression-review of baculovirus biotechnology. Environ Health Perspect 1995;103:756-9.
- Luckow VA, Summers MD. Trends in the development of baculovirus expression vectors. Biotechnology 1988;6:47-55.
- Beljelarskaya SN. A baculovirus expression system for insect cells. Mol Biol 2002;36:281-92.
- Ikonomou L, Schneider YJ, Agathos SN. Insect cell culture for industrial production of recombinant proteins. Appl Microbiol Biotechnol 2003;62:1-20.
- Cha HJ, Pham MQ, Rao G, Bentley WE. Expression of green fluorescent protein in insect larvae and its application for heterologous protein production. Biotechnol Bioeng 1997;56:239-47.
- Cha HJ, Dalal NG, Pham MQ, Vakharia VN, Rao G, Bentley WE. Insect larval expression process is optimized by generating fusions with green fluorescent protein. Biotechnol Bioeng 1999;65:316-24.
- Lihoradova OA, Bachurina EY, Azimova SS. Biosynthesis of the recombinant middle surface antigen of the human hepatitis B virus in silkworm larvae. Mol Biol 2004;38:603-7.
- Maeda S. Expression of foreign genes in insects using baculovirus vectors. Ann Rev Entomol 1989;34:351-72.
- Palhan VB, Sumathy S, Gopinathan KP. Baculovirus mediated high-level expression of luciferase in silkworm cells and larvae. Biotechniques 1995;19:97-104.
- Medin JA, Hunt L, Gathy K, Evans RK, Coleman MS. Efficient, low-cost protein factories: expression of human adenosine deaminase in baculovirus-infected insect larvae. Proc Natl Acad Sci USA 1990;87:2760-4.
- Casal JI. Use of parvovirus-like particles for vaccination and induction of multiple immune responses. Biotechnol Appl Biochem 1999;29:141-50.
- Lenz P, Day PM, Pang YYS, Frye SA, Jensen PN, Lowy DR, et al. Papillomavirus-like particles induce acute activation of dendritic cells. J Immunol 2001;166:5346-55.
- Schiller JT, Lowy DR. Papillomavirus-like particle based vaccines: cervical cancer and beyond. Expert Opin Biol Ther 2001;1:571-81.
- Roy P. Genetically engineered particulate virus-like structures and their use as vaccine delivery systems. Intervirology 1996;39:62-71.
- Xiang J, Wunschmann S, George SL, Klinzman D, Schmidt WN, LaBrecque DR, et al. Recombinant hepatitis C virus-like particles expressed by baculovirus: utility in cell-binding and antibody detection assays. J Med Virol 2002;68:537-43.
- Touze A, Bousarghin L, Ster C, Combita AL, Roingeard P, Coursaget P. Gene transfer using human polyomavirus BK virus-like particles expressed in insect cells. J Gen Virol 2001;82:3005-9.
- Tegerstedt K, Andreasson K, Vlastos A, Hedlund KO, Dalianis T, Ramqvist T. Murine pneumotropic virus VP1 virus-like particles (VLPs) bind to several cell types independent of sialic acid residues and do not serologically cross react with murine polyomavirus VP1 VLPs. J Gen Virol 2003;84:3443-52.
- Luo L, Li Y, Cannon P, Kim S, Kang CY. Chimeric gag-V3 virus-like particles of human immunodeficiency virus induce virus-neutralizing antibodies. Proc Natl Acad Sci USA 1992;89:10527-31.
- Tatman JD, Preston VG, Nicholson P, Elliott RM, Rixon FJ. Assembly of herpes simplex virus type 1 capsids using a panel of recombinant baculoviruses. J Gen Virol 1994;75:1101-13.
- Volpers C, Schirmacher P, Streeck RE, Sapp M. Assembly of the major and the minor capsid protein of human papillomavirus type 33 into virus-like particles and tubular structures in insect cells. Virology 1994;200:504-12.
- Gillock ET, Rottinghaus S, Chang D, Cai X, Smiley SA, An K, et al. Polyomavirus major capsid protein VP1 is capable of packaging cellular DNA when expressed in the baculovirus system. J Virol 1997;71:2857-65.
- Kajigaya S, Fujii H, Field A, Anderson S, Rosenfeld S, Anderson L, et al. Self-assembled B19 parvovirus capsids, produced in a baculovirus system, are antigenically and immunogenically similar to native virions. Proc Natl Acad Sci USA 1991;88:4646-50.
- Hu YC, Bentley WE, Edwards GH, Vakharia VN. Chimeric infectious bursal disease virus-like particles expressed in insect cells and purified by immobilized metal affinity chromatography. Biotechnol Bioeng 1999;63:721-9.
- Hu YC, Hsu TA, Huang JH, Ho MS, Ho YC. Formation of enterovirus-like particle aggregates by recombinant baculoviruses co-expressing P1 and 3CD in insect cells. Biotechnol Lett 2003;25:919-25.
- Ho Y, Lin PH, Liu CYY, Lee SP, Chao YC. Assembly of human severe acute respiratory syndrome coronavirus-like particles. Biochem Biophys Res Commun 2004;318:833-8.
- Mortola E, Roy P. Efficient assembly and release of SARS coronavirus-like particles by a heterologous expression system. FEBS Lett 2004;576:174-8.
- Tsao E, Mason M, Cacciuttolo M, Bowen S, Wassweman G. Production of parvovirus B19 vaccine in insect cells co-infected with double baculovirus. Biotechnol Bioeng 1996;49:130-8.
- Wang C, Olsen J, Zhang Y, Barklis E. Assembly of HIV GAG-βgalactosidase fusion proteins into virus particles. Virology 1994;200:524-34.
- Conner M, Zarley C, Hu B, Parsons S, Drabinski D, Greiner S, et al. Virus-like particles as a rotavirus subunit vaccine. J Infect Dis 1996;174:S88-S92.
- Gerhardt E, Bruss V. Phenotypic mixing of rodent but not avian hepadnavirus surface proteins into human hepatitis B virus particles. J Virol 1995;69:1201-8.
- Jarvis DL, Fleming J, Kovacs GR, Summers MD, Guarino LA. Use of early baculovirus promoters for continuous expression and efficient processing of foreign gene products in stably transformed lepidopteran cells. Biotechnology 1990;8:950-55.
- Ho Y, Lo HR, Lee TC, Wu CP, Chao YC. Enhancement of correct protein folding in vivo by a non-lytic baculovirus. Biochem J 2004;382:695-702.
- Raju TS, Lerner L, O’Connor JV. Glycopinion: biological significance and methods for the analysis of complex carbohydrates of recombinant glycoproteins. Biotechnol Appl Biochem 1996;24:191-4.
- Hollister JR, Shaper JH, Jarvis DL. Stable expression of mammalian beta 1,4-galactosyltransferase extends the N-glycosylation pathway in insect cells. Glycobiology 1998;8:473-80.
- Hollister JR, Jarvis DL. A stably-transformed insect cell line engineered to support asialoglycoprotein production by baculo-virus expression vectors. Glycobiology 1999;9:1124.
- Altmann F, Staudacher E, Wilson IBH, Marz L. Insect cells as hosts for the expression of recombinant glycoproteins. Glycoconj J 1999;16:109-23.
- Jarvis DL. Developing baculovirus-insect cell expression systems for humanized recombinant glycoprotein production. Virology 2003;310:1-7.
- Jarvis DL, Aumiller JJ, Hollister JR, Kawar ZS, Pilon J, Vadaie N. Analysis and manipulation of the protein N-glycosylation pathway in the baculovirus-insect cell system. Glycobiology 2004;14:1059-60.
- Laprise M-H, Grondin F, Dubois CM. Enhanced TGFβ1 maturation in high five cells coinfected with recombinant baculovirus encoding the convertase furin/pace: improved technology for the production of recombinant proproteins in insect cells. Biotechnol Bioeng 1998;58:85-91.
- Volkman LE, Goldsmith PA. In vitro study of Autographa californica nuclear polyhedrosis virus interaction with nontarget vertebrate host cells. Appl Environ Microbiol 1983;45:1085-93.
- Carbonell LF, Klowden MJ, Miller LK. Baculovirus-mediated expression of bacterial genes in dipteran and mammalain cells. J Virol 1985;56:153-60.
- Hofmann C, Sandig V, Jennings G, Rudolph M, Schlag P, Strauss M. Efficient gene-transfer into human hepatocytes by baculovirus vectors. Proc Natl Acad Sci USA 1995; 92: 10 099–103.
- Boyce FM, Bucher NLR. Baculovirus-mediated gene transfer into mammalian cells. Proc Natl Acad Sci USA 1996;93:2348-52.
- Shoji I, Aizaki H, Tani H, Ishii K, Chiba T, Saito I, et al. Efficient gene transfer into various mammalian cells, including non-hepatic cells, by baculovirus vectors. J Gen Virol 1997;78:2657-64.
- Kost TA, Condreay JP. Recombinant baculoviruses as mammalian cell gene delivery vectors. Trends Biotechnol 2002;20:173-80.
- Leisy DJ, Lewis TD, Leong JAC, Rohrmann GF. Transduction of cultured fish cells with recombinant baculoviruses. J Gen Virol 2003;84:1173-8.
- van Loo ND, Fortunati E, Ehlert E, Rabelink M, Grosveld F, Scholte BJ. Baculovirus infection of nondividing mammalian cells: mechanisms of entry and nuclear transport of capsids. J Virol 2001;75:961-70.
- Sarkis C, Serguera C, Petres S, Buchet D, Ridet JL, Edelman L, et al. Efficient transduction of neural cells in vitro and in vivo by a baculovirus-derived vector. Proc Natl Acad Sci USA 2000;97:14638-43.
- Ma L, Tamarina N, Wang Y, Kuznetsov A, Patel N, Kending C, et al. Baculovirus-mediated gene transfer into pancreatic islet cells. Diabetes 2000;49:1986-91.
- Ho YC, Chen HC, Wang KC, Hu YC. Highly efficient baculovirus-mediated gene transfer into rat chondrocytes. Biotechnol Bioeng 2004;88:643-51.
- Wagle M, Jesuthasan S. Baculovirus-mediated gene expression in zebrafish. Marine Biotechnol 2003;5:58-63.
- Condreay JP, Witherspoon SM, Clay WC, Kost TA. Transient and stable gene expression in mammalian cells transduced with a recombinant baculovirus vector. Proc Natl Acad Sci USA 1999;96:127-32.
- Cheng T, Xu CY, Wang YB, Chen M, Wu T, Zhang J, et al. A rapid and efficient method to express target genes in mammalian cells by baculovirus. World J Gastroenterol 2004;10:1612-18.
- Lo HR, Chou CC, Wu TY, Yuen JP, Chao YC. Novel baculovirus DNA elements strongly stimulate activities of exogenous and endogenous promoters. J Biol Chem 2002;277:5256-64.
- Venkaiah B, Viswanathan P, Habib S, Hasnain SE. An additional copy of the homologous region (hr1) sequence in the Autographa californica multinucleocapsid polyhedrosis virus genome promotes hyperexpression of foreign genes. Biochemistry 2004;43:8143-51.
- Viswanathan P, Venkaiah B, Kumar MS, Rasheedi S, Vrati S, Bashyam MD, . The homologous region sequence (hr1) of multinucleocapsid polyhedrosis virus can enhance transcription from non-baculoviral promoters in mammalian cells. J Biol Chem 2003; 278: 52 564–71.
- Pijlman GP, de Vrij J, van den End FJ, Vlak JM, Martens DE. Evaluation of baculovirus expression vectors with enhanced stability in continuous cascaded insect-cell bioreactors. Biotechnol Bioeng 2004;87:743-53.
- Pijlman GP, van den Born E, Martens DE, Vlak JM. Autographa californica baculoviruses with large genomic deletions are rapidly generated in infected insect cells. Virology 2001;283:132-8.
- Hu YC, Tsai CT, Chang YJ, Huang JH. Enhancement and prolongation of baculovirus-mediated expression in mammalian cells: Focuses on strategic infection and feeding. Biotechnol Prog 2003;19:373-9.
- Tani H, Limn CK, Yap CC, Onishi M, Nozaki M, Nishimune Y, et al. In vitro and in vivo gene delivery by recombinant baculo-viruses. J Virol 2003;77:9799-808.
- Hsu CS, Ho YC, Wang KC, Hu YC. Investigation of optimal transduction conditions for baculovirus-mediated gene delivery into mammalian cells. Biotechnol Bioeng 2004;88:42-51.
- Ho YC, Chung YC, Hwang SM, Wang KC, Hu YC. Transgene expression and differentiation of baculovirus-transduced human mesenchymal stem cells. J Gene Med 2005. in press.
- Gao R, McCormick CJ, Arthur MJP, Ruddle R, Oakley F, Smart DE, et al. High efficiency gene transfer into cultured primary rat and human hepatic stellate cells using baculovirus vectors. Liver 2002;22:15-22.
- Detrait ER, Bowers WJ, Halterman MW, Giuliano RE, Bennice L, Federoff HJ, et al. Reporter gene transfer induces apoptosis in primary cortical neurons. Mol Ther 2002;5:723-30.
- Liu HS, Jan MS, Chou CK, Chen PH, Ke NJ. Is green fluorescent protein toxic to the living cells? Biochem Biophys Res Commun 1999;260:712-17.
- Wang YC, Huang CF, Tung SF, Lin YS. Competition with TATA box-binding protein for binding to the TATA box implicated in human cytomegalovirus IE2-mediated transcriptional repression of cellular promoters. DNA Cell Biol 2000;19:613-19.
- Dee KU, Shuler ML. A mathematical model of the trafficking of acid-dependent enveloped viruses: application to the binding, uptake, and nuclear accumulation of baculovirus. Biotechnol Bioeng 1997;54:468-90.
- Duisit G, Saleun S, Douthe S, Barsoum J, Chadeuf G, Moullier P. Baculovirus vector requires electrostatic interactions including heparan sulfate for efficient gene transfer in mammalian cells. J Gene Med 1999;1:93-102.
- Hofmann C, Lehnet W, Strauss M. The baculovirus system for gene delivery into hepatocytes. Gene Ther Mol Biol 1998;1:231-9.
- Tani H, Nishijima M, Ushijima H, Miyamura T, Matsuura Y. Characterization of cell-surface determinants important for baculovirus infection. Virology 2001;279:343-53.
- Bilello JP, Cable EE, Myers RL, Isom HC. Role of paracellular junction complexes in baculovirus-mediated gene transfer to nondividing rat hepatocytes. Gene Ther 2003;10:733-49.
- Barsoum J, Brown R, McKee M, Boyce FM. Efficient transduction of mammalian cells by a recombinant baculovirus having the vesicular stomatitis virus G glycoprotein. Hum Gene Ther 1997;8:2011-8.
- Kukkonen SP, Airenne KJ, Marjomaki V, Laitinen OH, Lehtolainen P, Kankaanpaa P, et al. Baculovirus capsid display: a novel tool for transduction imaging. Mol Ther 2003;8:853-62.
- Boublik Y, Di Bonito P, Jones IM. Eukaryotic virus display: engineering the major surface glycoprotein of the Autographa californica nuclear polyhedrosis virus (AcNPV) for the presentation of foreign proteins on the virus surface. Biotechnology 1995;13:1079-84.
- Raty JK, Airenne KJ, Marttila AT, Marjomaki V, Hytonen VP, Lehtolainen P, et al. Enhanced gene delivery by avidin-displaying baculovirus. Mol Ther 2004;9:282-91.
- Mottershead DG, Alfthan K, Ojala K, Takkinen K, Oker-Blom C. Baculoviral display of functional scFv and synthetic IgG-binding domains. Biochem Biophys Res Commun 2000;275:84-90.
- Ojala K, Mottershead DG, Suokko A, Oker-Blom C. Specific binding of baculoviruses displaying gp64 fusion proteins to mammalian cells. Biochem Biophys Res Commun 2001;284:777-84.
- Ernst W, Grabherr R, Wegner D, Borth N, Grassauer A, Katinger H. Baculovirus surface display: construction and screening of a eukaryotic epitope library. Nucleic Acids Res 1998;26:1718-23.
- Cheshenko N, Krougliak N, Eisensmith RC, Krougliak VA. A novel system for the production of fully deleted adenovirus vectors that does not require helper adenovirus. Gene Ther 2001;8:846-54.
- Sollerbrant K, Elmen J, Wahlestedt C, Acker J, Leblois-Prehaud H, Latta-Mahieu M, et al. A novel method using baculovirus-mediated gene transfer for production of recombinant adeno-associated virus vectors. J Gen Virol 2001;82:2051-60.
- Ramos L, Kopec LA, Sweitzer SM, Fornwald JA, Zhao HZ, McAllister P, et al. Rapid expression of recombinant proteins in modified CHO cells using the baculovirus system. Cytotechnology 2002;38:37-41.
- Wang KC, Wu JC, Chung YC, Ho YC, Chang MD, Hu YC. Baculo-virus as a highly efficient gene delivery vector for the expression of hepatitis delta virus antigens in mammalian cells. Biotechnol Bioeng 2005;89:464-73.
- Chen YH, Wu JC, Wang KC, Chiang YW, Lai CW, Chung YC, et al. Baculovirus-mediated production of HDV-like particles in BHK cells using a novel oscillating bioreactor. J Biotechnol 2005. in press.
- Clay WC, Condreay JP, Moore LB, Weaver SL, Watson MA, Kost TA, et al. Recombinant baculoviruses used to study estrogen receptor function in human osteosarcoma cells. Assay Drug Dev Technol 2003;1:801-10.
- Jenkinson S, McCoy DC, Kerner SA, Ferris RG, Lawrence WK, Clay WC, et al. Development of a novel high-throughput surrogate assay to measure HIV envelope/CCR5/CD4-mediated viral/cell fusion using BacMam baculovirus technology. J Biomol Screen 2003;8:463-70.
- Delaney WE, Isom HC. Hepatitis B virus replication in human HepG2 cells mediated by hepatitis B virus recombinant baculo-virus. Hepatology 1998;28:1134-46.
- Fipaldini C, Bellei B, La Monica N. Expression of hepatitis C virus cDNA in human hepatoma cell line mediated by a hybrid baculovirus-HCV vector. Virology 1999;255:302-11.
- Delaney WE, Miller TG, Isom HC. Use of the hepatitis B virus recombinant baculovirus-HepG2 system to study the effects of (-)-beta-2',3'-dideoxy-3'-thiacytidine on replication of hepatitis B virus and accumulation of covalently closed circular DNA. Antimicrob Agents Chemother 1999;43:2017-26.
- Delaney WE, Edwards R, Colledge D, Shaw T, Torresi J, Miller TG, et al. Cross-resistance testing of antihepadnaviral compounds using novel recombinant baculoviruses which encode drug-resistant strains of hepatitis B virus. Antimicrob Agents Chemother 2001;45:1705-13.
- McCormick CJ, Rowlands DJ, Harris M. Efficient delivery and regulable expression of hepatitis C virus full-length and minigenome constructs in hepatocyte-derived cell lines using baculovirus vectors. J Gen Virol 2002;83:383-94.
- Merrihew RV, Clay WC, Condreay JP, Witherspoon SM, Dallas WS, Kost TA. Chromosomal integration of transduced recombinant baculovirus DNA in mammalian cells. J Virol 2001;75:903-9.
- Hofmann C, Strauss M. Baculovirus-mediated gene transfer in the presence of human serum or blood facilitated by inhibition of the complement system. Gene Ther 1998;5:531-6.
- Airenne KJ, Hiltunen MO, Turunen MP, Turunen AM, Laitinen OH, Kulomaa MS, et al. Baculovirus-mediated periadventitial gene transfer to rabbit carotid artery. Gene Ther 2000;7:1499-1504.
- Lehtolainen P, Tyynela K, Kannasto J, Airenne KJ, Yla-Herttuala S. Baculoviruses exhibit restricted cell type specificity in rat brain: a comparison of baculovirus- and adenovirus-mediated intracerebral gene transfer in vivo. Gene Ther 2002;9:1693-9.
- Huser A, Rudolph M, Hofmann C. Incorporation of decay-accelerating factor into the baculovirus envelope generates complement-resistant gene transfer vectors. Nature Biotechnol 2001;19:451-5.
- Pieroni L, Maione D, La Monica N. In vivo gene transfer in mouse skeletal muscle mediated by baculovirus vectors. Hum Gene Ther 2001;12:871-81.
- Bilello JP, Delaney WE, Boyce FM, Isom HC. Baculovirus entry into nondividing hepatocytes is enhanced by transient disruption of intercellular junctions. Hepatology 2001;34:834.
- Barsoum J. Concentration of recombinant baculovirus by cation-exchange chromatography. Biotechniques 1999;26:834-40.
- Hu YC, Tsai CT, Chung YC, Lu JT, Hsu JT. Generation of chimeric baculovirus with histidine-tags displayed on the envelope and its purification using immobilized metal affinity chromatography. Enzyme Microb Technol 2003;33:445-52.
- Sandig V, Hofmann C, Steinert S, Jennings G, Schlag P, Strauss M. Gene transfer into hepatocytes and human liver tissue by baculovirus vectors. Hum Gene Ther 1996;7:1937-45.
- Gronowski AM, Hilbert DM, Sheehan KCF, Garotta G, Schreiber RD. Baculovirus stimulates antiviral effects in mammalian cells. J Virol 1999;73:9944-51.
- Beck NB, Sidhu JS, Omiecinski CJ. Baculovirus vectors repress phenobarbital-mediated gene induction and stimulate cytokine expression in primary cultures of rat hepatocytes. Gene Ther 2000;7:1274-83.
- Abe T, Takahashi H, Hamazaki H, Miyano-Kurosaki N, Matsuura Y, Takaku H. Baculovirus induces an innate immune response and confers protection from lethal influenza virus infection in mice. J Immunol 2003;171:1133-9.
- Aoki H, Sakoda Y, Jukuroki K, Takada A, Kida H, Fukusho A. Induction of antibodies in mice by a recombinant baculovirus expressing pseudorabies virus glycoprotein B in mammalian cells. Vet Microbiol 1999;68:197-207.
- Facciabene A, Aurisicchio L, La Monica N. Baculovirus vectors elicit antigen-specific immune responses in mice. J Virol 2004;78:8663-72.
- Tami C, Farber M, Palma EL, Taboga O. Presentation of antigenic sites from foot-and-mouth disease virus on the surface of baculovirus and in the membrane of infected cells. Arch Virol 2000;145:1815-28.
- Yoshida S, Kondoh D, Arai E, Matsuoka H, Seki C, Tanaka T, et al. Baculovirus virions displaying Plasmodium berghei circumsporozoite protein protect mice against malaria sporozoite infection. Virology 2003;316:161-70.
- Baumert TF, Ito S, Wong DT, Liang TJ. Hepatitis C virus structural proteins assemble into viruslike particles in insect cells. J Virol 1998;72:3827-36.
- O’Reilly D, Miller L, Luckow V. In: Baculovirus expression vectors: a laboratory manual. New York: WH Freeman and Co; 1992.