Melatonin attenuates 1-methyl-4-phenylpyridinium-induced PC12 cell death
Introduction
1-Methyl-4-phenyl-1,2,3,6-tetrahydropyridine (MPTP)-mediated selective damage to dopaminergic neurons of the nigrostriatal pathway has been widely used as a model of Parkinson’s disease (PD)[1–3]. MPTP is a lipophilic molecule that is able to cross the blood-brain barrier. This cytotoxin has provided important information on the potential mechanisms of PD. It induces toxicity through its conversion of astrocytes to 1-methyl-4-phenylpyridinium (MPP+) in the reaction catalyzed by monoamine oxidase type B (MAO-B)[4]. MPP+ is selectively transported into the dopaminergic neurons through a high-affinity dopamine (DA) transporter and accumulates in the neuronal mitochondria, where it disrupts cellular respiration inhibiting complex I of the electron transport chain, depletes adenosine triphosphate (ATP) in the mitochondria[5,6], increases sensitivity to oxidative attack, and eventually causes apoptotic or necrotic neuronal cell death. It is reported that the deleterious effects of MPTP are results of the generation of oxygen free radicals either during oxidation of MPTP to MPP+, or during electron transport chain disruption by MPP+. In addition, MPP+ might induce the release of DA, which in turn could generate free radicals from auto-oxidation[7,8]. Although it has been found that the accumulation of MPP+ in dopaminergic neurons is a critical step for DA neurotoxicity, the exact molecular mechanisms underlying MPP+-induced neuronal death have not been clear. Thus, elucidating the molecular basis of the cell death triggered by MPP+ could provide valuable insight into the pathogenesis of PD.
Melatonin is an indole hormone produced primarily in the pineal gland and the retina. It is classically known to be involved in the regulation of circadian and seasonal rhythms and sleep-inducing properties. In 1993, it was first reported by Tan et al as an efficient endogenous antioxidant[9]. Since then, evidence is shown that this molecule is one of the best physiological antioxidants. Different from other potent antioxidants, melatonin is soluble in both lipids and water, allowing itself to easily enter cells or pass the blood-brain barrier. So melatonin is available to all nerve cell compartments and shows high levels, especially in the nucleus and mitochondria. Recently, it has been proposed that the most important function of this hormone is scavenging harmful free radicals, especially hydroxyl radicals. Tan et al[9] made the unexpected observation that melatonin was much more efficient than the important intracellular antioxidant glutathione (GSH) in scavenging ·OH. Mayo et al[10] also reported that melatonin regulated the mRNA of antioxidant enzymes in dopaminergic cells after addition of the neurotoxin 6-OHDA, which might indicate a neuroprotective mechanism. However, intracellular effects of melatonin are largely unknown at present, in contrast to well-studied antioxidant activity.
PC12 cell is a clonal cell line of rat pheochromocytoma cells that respond to nerve growth factor by extending neuritis and acquiring the appearance of neurons. Cells with and without nerve growth factor treatment synthesize, store, secrete, and take up dopamine by similar processes to those of dopaminergic neurons[11], and express DA transporter[12]. Although they are not true brain DA neurons, PC12 cells have been used extensively as an experimental system for the study of various aspects of dopaminergic neurons and neurotoxins[13–15]. In this study, we used PC12 cells as a model cell line of DA-containing neurons and investigated the effects of melatonin on MPP+-induced cell death.
Materials and methods
Materials Dulbecco’s modified Eagle’s medium (DMEM), fetal calf-serum and benzylpenicillin/streptomycin were pruchased from GIBCO (Langley, OK, USA ). Poly-L-lysine, trypsin, dimethylsulfoxide (Me2SO), diaminobenzidine (DAB), 3-(4,5-Dimethylthiazol-2-yl)-2,5-diphenyltetrazolium bromide (MTT) were purchased from Sigma (St Louis, MO, USA). MPP+-iodine was purchased from Sigma-Aldrich. Rabbit polyclonal anti-tyrosine hydroxylase (TH) antiserum was purchased from Chemicon (Tenecula, CA, USA). Biotinylated goat anti-rabbit IgG, horseradish peroxidase-conjugated secondary antibodies, ABC kit were purchased from Santa Cruz Biotechnology (Santa Cruz, CA, USA). Melatonin was purchased from Sigma and dissolved in absolute ethanol before being diluted with saline. All other chemicals were of analytical grade and commercially available.
PC12 cells culture and treatment PC12 cells were obtained from the Shanghai Cell Research Institute and cultured at a density of 1×104 cells/cm2 on poly-L-lysine-coated plates. Cells were cultured in high-glucose DMEM supplemented with heat-inactivated 10% fetal bovine serum, 5% horse serum, benzylpenicillin 100 kU/L, and streptomycin 100 mg/L. They were grown at 37 ºC in a humidified atmosphere containing 5% CO2 and subcultured every 2–3 d at approximately 70%–80% confluence. Cells were subsequently switched to 5% fetal calf serum low glucose, serum-free high glucose, and serum-free low glucose DMEM medium, containing various experimental reagents, and further incubated for the indicated intervals. Drugs and other agents were dissolved in Me2SO, which was added at a maximum ratio of 1:1000 to the culture media. Cultures were used for no more than 20 passages. Cell viability experiments were performed in 96-well plates, 8 times, using 2–3 replicates per treatment. In dose-response studies, cells were treated with MPP+ (0–500 μmol/L) for 48 h with or without melatonin (0.1–1000 μmol/L). In time course studies, cells were exposed to MPP+ (200 μmol/L) for 0, 24, 48, 72, and 96 h with or without melatonin 250 μmol/L. The cells were preincubated with melatonin for 1 h before treatment with MPP+. The final concentration of ethanol per well did not exceed 0.01% for all melatonin concentrations used. The vehicle-treated control cultures received an equal amount of ethanol (0.01%). For cytotoxicity, cells were seeded on plates previously coated with poly-L-lysine. Untreated cells were used as control. For TH immunocytochemistry, the cells were subcultured for 48 h before use of 22-mm coverslips (Fisher Scientific, Pittsburgh, PA, USA), coated with poly-L-lysine in 35-mm culture dishes (Fisher Scientific).
MTT assay Cell viability was measured by MTT assay[16], which was based on the conversion of MTT to formazan crystals by mitochondrial dehydrogenase. Cells were plated at a density of 2×104 cells in 96-well dishes in 10% serum high-glucose DMEM medium for 2 d, and switched to 5% fetal calf serum low glucose, serum-free high glucose, and serum-free low glucose DMEM medium containing the experimental reagents. Four hours before the desired end point, MTT (5 g/L in PBS, final concentration 0.5 g/L) was added at 1/10 culture volume and cells were returned to the incubator. After the end point, culture medium was removed, and 100 µL of Me2SO was added to each well to dissolve formazan. Absorbance (OD value) was measured at 570 nm in a microplate reader (Bio-Rad model 550). Cell viability was expressed by OD value.
LDH release assay[15] Cytotoxicity of MPP+ was evaluated by colorimetric assay based on the measurement of LDH activity. Briefly, after cells were treated with MPP+, an aliquot of medium was taken and centrifuged at 250×g for 5 min. Supernatant (10 µL) was added to the LDH buffer [lactic acid 50 mmol/L/Tris (pH 8.9) 0.1 mol/L and NAD+ (the oxidized form of nicotinamide-adenine dinucleotide) 4.6 g/L] to a total volume of 200 µL. Intracellular LDH content was determined by lysing the cells with Triton X-100 detergent. Spectrophotometric analysis was performed at room temperature (20–24 ºC) using a Bio-Rad microplate reader measuring optical density at 340 nm in both the medium and lysis buffer. LDH release values were calculated by subtracting the background reading (media only) from the observed reading. LDH leakage was expressed as a percentage of the total LDH release (extracellular LDH extracellular+intracellular LDH).
Tyrosine hydroxylase (TH) immunocytochemistry TH activity was identified using the streptavidin-biotin-peroxidase complex. Cultured in poly-L-lysine coated coverslips, PC12 cells (2×104) were treated with MPP+ 200 µmol/L with or without melatonin in serum-free low glucose medium. Melatonin was added 1 h before MPP+ treatment. Cultures were incubated at 37 ºC, 5% CO2 for a further 48 h, PC12 cells were washed with phosphate-buffered saline 0.1 mol/L (PBS, pH 7.4) and then fixed in 4% paraformaldehyde (pH 7.4) in PBS for at least 1 h. PC12 cells were incubated with 1% H2O2 and 30% methanol in 0.3 mol/L PBS to block endogenous peroxidases. The sections were rinsed three times in PBS and incubated successively with 1) normal sheep serum (20% in PBS) to reduce nonspecific binding for 30 min in a moisture -saturated chamber at 37 ºC; 2) antityrosine hydroxylase rabbit antiserum at 1/300 overnight in a moisture-saturated chamber at 4 ºC for 48 h; 3) biotinylated anti-rabbit IgG at 1:200 for 30 min in a moisture saturated-chamber at room temperature (20–24 ºC); and 4) streptavidin-peroxidase complex at 1:100 for 30 min in a moisture-saturated chamber at room temperature (20–24 ºC). Sections were rinsed with PBS after each step (3×5 min). In step 2, control sections were incubated with an equal volume of PBS, rather than antiserum, as negative controls. The streptavidin-biotin-peroxidase activity was revealed using 3,3´-diamino-benzidine tetrachloride (DAB, 0.5 g/L), in the presence of 0.03% H2O2 in PBS for 5 min. Coverslips were washed with PBS and dehydrated through ethanol. Finally, sections were mounted on gelatin-coated slides, air-dried, dehydrated, and coverslipped with permount. Immunocytochemical staining was analyzed at 400×magnification on an Olympus inverted phase-contrast microscope. Micrographs were taken with a camera mounted on the phototube using a Kodak film.
Statistical analysis Statistical analysis was performed using t-test for paired data. Data were expressed as the mean±SD. P<0.05 was considered to be statistically significant.
Results
Effect of MPP+ on PC12 cells viability The reduction was markedly decreased after PC12 cultures were exposed to MPP+ (200 µmol/L) in low glucose condition. MTT reduction was significantly decreased after MPP+ treatment in serum low glucose condition. The percentage of cell viability was approximately 50%, 44%, and 30% at 48, 72, and 96 h, respectively. In serum-free low glucose condition, the percentages of cell viability were 85.1%, 75.4%, 64.9%, 28.1%, and 9% at 12, 24, 48, 72, and 96 h after MPP+ treatment, respectively. However, when cells were exposed to MPP+ 200 µmol/L in high glucose condition, no significant cell death appeared compared to control. MPP+ induced a slight decrease in cell viability at 25 and 50 µmol/L, and more significantly at 100 µmol/L in the serum-free low glucose condition. In the 5% serum low glucose condition, the cell viabilities after MPP+ (25, 50, and 100 µmol/L) treatment had no obvious changes compared with control, and significant cell death was observed at MPP+ 200 µmol/L. Therefore, MPP+ induced dose-dependent cell death in the low glucose condition. However, in the high glucose condition, no cell death was seen for all concentrations of MPP+. We found that supplementing glucose increased the number of surviving cells for all concentrations of MPP+.
Melatonin promotes PC12 survial after MPP+ treatment The percentage of viable cells in serum-free low glucose condition with low dose melatonin (0.1, 1, and 10 µmol/L) did not change after treatment with MPP+. However, when the cells were preincubated with melatonin (100, 250, 500, 1000 µmol/L), MPP+-induced cell toxicity was significantly attenuated. Surviving PC12 cells were significantly increased from 53% in MPP+ to 80% when melatonin was added. Melatonin was protective against MPP+ toxicity in PC12 cells. Melatonin at 500 and 1000 µmol/L showed similar protection as 250 µmol/L melatonin, although a tendency to increase effects with higher doses was observed, indicating that the dose of 250 µmol/L might be close to the maximal effect (Figure 1).
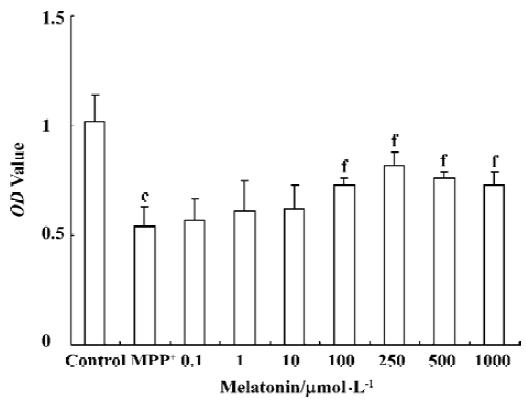
The cultures were preincubated with melatonin (250 μmol/L) for 1 h and then exposed to MPP+ (200 μmol/L) for 0, 12, 24, 48, 72, and 96 h in serum or serum-free low glucose condition. At the end of each time period, cell viability was assessed by MTT reduction. In serum-free low glucose condition, cell viability was decreased at 24, 48, 72, and 96 h to 75.4%, 64.9%, 28.1%, and 9% respectively, after MPP+ treatment (200 μmol/L). Melatonin (250 μmol/L) significantly increased survival of cells exposed to MPP+ (200 μmol/L), cell viability was 78.1%, 58.8%, and 31.6% at 48, 72, and 96 h, respectively. In 5% serum low glucose condition, melatonin also significantly enhanced survival of cells exposed to MPP+ (P<0.05) (Figure 2).
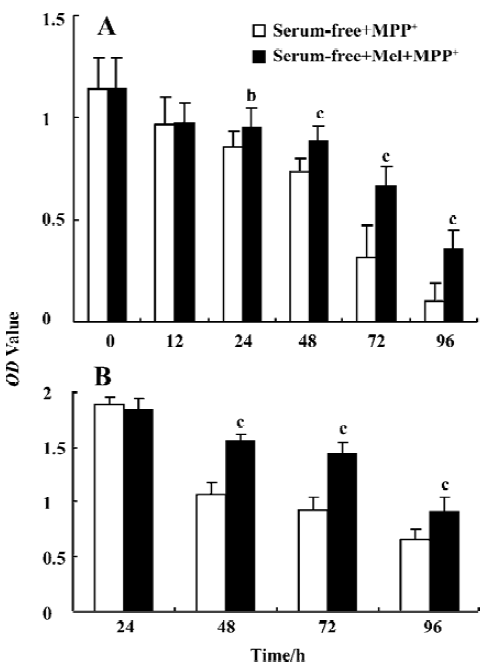
LDH release MPP+ caused membrane damage, which was determined by the release of the 24 kDa cytosolic enzyme LDH into the culture medium. The level of LDH into the culture medium was found to be 2-fold lower after MPP+ treatment in 5% serum condition than after treatment with MPP+ in serum-free medium for 48 h (16.3%±1.3%, and 29.4%±1.4%, respectively). MPP+ treatment for 12 h at 200 µmol/L did not affect cell viability as revealed by lactate LDH (data not shown). However, after PC12 cells were incubated for 48 h with MPP+, in either serum or serum-free condition, there was a significant increase in LDH leakage into the medium. LDH levels were further increased after MPP+ treatment for 72 and 96 h. Melatonin 250 µmol/L significantly abolished the increment in LDH leakage produced by MPP+ (Figure 3).
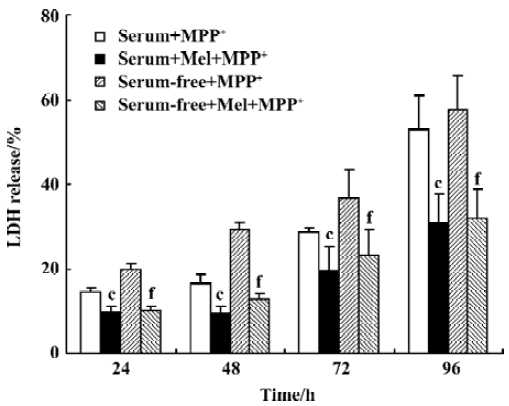
TH immunocytochemistry Tyrosine hydroxylase immunocytochemistry studies showed loss of the TH immunoreactivity in the PC12 cells after MPP+ treatment. Addition of melatonin 250 µmol/L significantly increased the survival of TH+ cells. The number of TH-immunoreactive cells after melatonin treatment was greater than that in MPP+-treated only cells, but less than that in control cells (Figure 4).
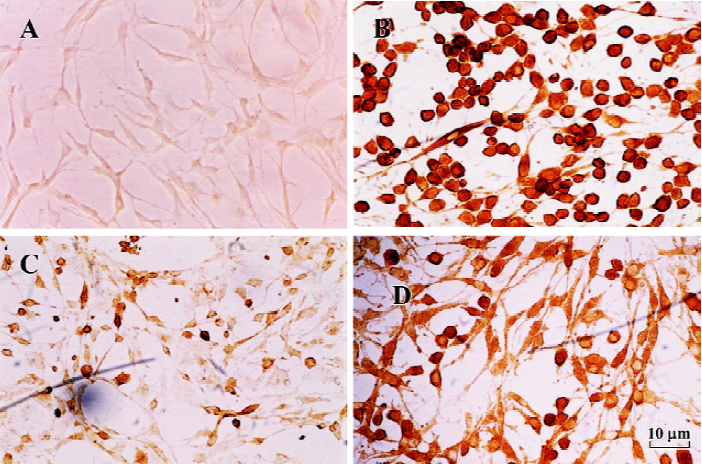
Discussion
An increasing body of evidence suggests that the cell energy path is an important target in the pathogenesis of neurodegenerative diseases. MPTP induces direct inhibition of complex I of the mitochondrial respiratory chain, which produces a large number of free radicals and energy crisis in the mitochondria. An excess of free radicals leads to mitochondrial damage, causing a failure of cellular energy metabolism followed by a secondary excitotoxicity. During our research, on the effect of melatonin on PC12 cell death induced by MPP+, we noticed that melatonin at low glucose condition had a neuroprotective effect. The neurotoxicity of MPP+ is widely acknowledged. In addition, we discovered that this neurotoxicity was dependent on the glucose level. MPP+ had obvious toxicity in low glucose condition but not in high glucose condition. Our results suggested that PC12 cells might be vulnerable to MPP+ in a low glucose condition but resistant to the injury induced by MPP+ in a high glucose condition. Basma et al[17] demonstrated that the toxicity of an MPTP derivative to PC12 cells was exacerbated by inhibiting glycolysis. This was consistent with our results. Indeed, increasing the concentration of glucose reduced MPP+-induced cytotoxicity at all MPP+ concentrations accordingly. Depletion of glucose in the medium limited the ability of the cells to drive energy from glycolysis and, furthermore, rapid depletion of limited ATP supply through inhibition of mitochondrial function by MPP+ resulted in cell death. At lower glucose concentrations, the depletion would occur more rapidly, which resulted in higher toxicities. At higher glucose concentrations, glycolysis was able to compensate longer for loss of ATP as a result of the MPP+-induced inhibition of mitochondrial respiration and hence attenuated cell death. Kutty et al[18] reported similar findings using the neuroblastomax glioma hybrid NG 108-15 neuronal cell culture system. Using leakage of LDH and release of adenine as indices of toxicity, they found that the death of cells exposed to MPTP, MPP+, or 2’Et-MPP+, and the depletion of ATP caused by the neurotoxins, was markedly reduced owing to high glucose concentration (25 µmol/L) in the medium. The enhanced susceptibility of the cells grown in low glucose medium to the toxic effects of the neurotoxins was, most likely, caused by the action of the toxins on the glucose-depleted cells rather than directly because of the depletion of glucose.
The conversion of dye MTT to formazan crystals in cells has been shown to be related to mitochondrial redox state and respiratory chain activity[19]. In the present study, the result of MTT reduction could indicate a great role for mitochondrial dysfunction and cellular energy in these models of cell death. The reason seems to be that energy compromise plays a large role in the progression of PD. It is well known that the brain consumes large quantities of oxygen relative to total body mass. MPP+ could induce PC12 cell death by depleting cellular levels of ATP through inhibition of complex I. Low glucose levels or the inhibition of glycolysis potentiates MPP+ toxicity, while supplementation with fructose reduces the toxicity[17,20]. The data in this study provided additional support for the hypothesis that MPP+ kill cells by inhibiting mitochondrial respiration, and that cell energy status was an important factor for MPP+ induced cell death. These observations could prove useful in both the formulation of an accurate cell culture model of PD-like neuro-degeneration and the elucidation of the molecular basis of MPP+ toxicity.
In the present study on the efficacy of melatonin to MPP+- treated PC12 cells in low glucose culture, melatonin increased cell viability according to the MTT and LDH release assay. Single dose treatment of PC12 cells with melatonin (250 µmol/L) induced a significant decrease in LDH release after 48 h compared with vehicle-treated cultures. TH immunocytochemistry was used to visualize catecholaminergic neurons and monitor MPP+-induced changes at the cellular level. As shown here, melatonin also protected PC12 cells against MPP+-induced morphological changes. These results indicate that melatonin possesses the remarkable ability to rescue PC12 cells from cell death in our experimental paradigms associated with MPP+. The present findings indicate that the protective effects of melatonin against MPP+ toxicity may be a result of, at least partially, its antioxidant ability and mitochondria protection. Therefore, supplemental melatonin could ameliorate the severity of neurodegenerative changes caused by free radicals and mitochondrial dysfunc-tion. However, this remains to be confirmed with further investigation.
Our study also showed indications for susceptible neuronal populations. As nerve cells exclusively use glucose as an energy source, increasing glucose concentration in susceptible neuronal populations might be an alternative approach to treat patients with neurodegenerative diseases. Further studies of the actions of melatonin on neurons might also provide a basis for the development of new therapeutic approach to overcome neurodegenerative diseases, such as PD.
References
- Heikkila RE, Hess A, Duvoisin RC. Dopaminergic neurotoxicity of 1-methyl-4-phenyl-1,2,5,6-tetrahydropyridine in mice. Science 1984;224:1451-3.
- Langston JW, Ballard P, Tetrud JW, Irwin I. Chronic parkinsonism in human due to a product of meperidine-analog synthesis. Science 1983;219:979-80.
- Yang SF, Wu Q, Sun AS, Huang XN, Shi JS. Protective effect and mechanism on Ginkgo biloba leaf extracts for Parkinson disease induced by 1-methyl-4-phenyl-1,2,3,6-tetrahydro-pyridine. Acta Pharmacol Sin 2001;22:1089-93.
- Singer TP, Castagnoli N Jr, Ramsay RR, Trevor AJ. Biochemical events in the development of parkinsonism induced by 1-methyl-4-phenyl-1,2,3,6-tetrahydropyridine. J Neurochem 1987;49:1-8.
- Nicklas WJ, Vyas I, Heikkila RE. Inhibition of NADH-linked oxidation in brain mitochondria by 1-methyl-pyridine-1,2,3,6-tetrahydro-pyridine. Life Sci 1985;36:2503-8.
- Mizuno Y, Suzuki K, Sone N, Saitoh T. Inhibition of mitochondrial respiration by 1-methyl-4-phenyl-1,2,3,6-tetrahydropyridine (MPTP) in mouse brain in vivo. Neurosci Lett 1988;91:349-53.
- Hasegawa E, Takeshige K, Oishi T, Murai Y, Minakami S. 1-methyl-4-phenylpyridinium (MPP+) induced NADH-dependent superoxide formation and enhances NADH-dependent lipid peroxidation in bovine heart submitochondrial particles. Biochem Biophys Res Commun 1990;170:1049-55.
- Rossetti ZL, Sotgiu A, Sharp DE, Hadjiconstantinou M, Zeff NH. 1-methyl-4-phenyl-1,2,3,6-tetrahydropyridine (MPTP) and free radicals in vitro. Biochem Pharmacol 1988;37:4573-84.
- Tan DX, Chen LD, Poeggeler B, Manchester LC, Reiter RJ. Melatonin: a potent endogenous hydroxyl radical scavenger. Endocrine J 1993;1:57-60.
- Mayo JC, Sainz RM, Uría H, Antolín I, Esteban MM, Rodríguez C. Melatonin prevents apoptosis induced by 6-hydroxydopamine in neuronal cells: implications for Parkinson’s disease. J Pineal Res 1998;24:179-92.
- Greene LA, Rein G. Release, storage and uptake of catecholamines by clonal cell line of nerve growth factor (NGF) responsive pheochromocytoma cells. Brain Res 1977;129:247-63.
- Kadota T, Yamaai T, Saito Y, Akita Y, Kawashima S, Moroi K. Expression of dopamine transporter at the tips of growing neuritis of PC12 cells. J Histochem Cytochem 1996;44:989-96.
- Desole MS, Sciola L, Delogu MR, Sircana S, Miqnel R, Miek E. Role of oxidative stress in the manganese and 1-methyl-4-(2´-ethylphenyl)-1,2,3,5-tetrahydropyridine-induced apoptosis in PC12 cells. Neurochem Int 1996;31:169-76.
- Hartley A, Stone JM, Heron C, Cooper JM, Schspira AHV. Complex I inhibitors induce dose-dependent apoptosis in PC12 cells. J Neurochem 1994;63:1987-90.
- Song W, Guan HJ, Zhu XZ, Chen ZL, Yin ML, Cheng XF. Protective effect of bilobalide against nitric oxide-induced neurotoxicity in PC12 cells. Acta Pharmacol Sin 2000;21:415-23.
- Mosmann T. Rapid colorimetric assay for cellular growth and survival: application to proliferation and cytotoxicity assays. J Immunol Methods 1983;65:55-63.
- Basma AN, Heikkila RE, Saporito MS, Philbert M, Geller HM, Nicklas WJ. 1-Methyl-4-(2´-ethylphenyl)-1,2,3,6-tetrahydropyridine-induced toxicity in PC12 cells is enhanced by preventing glycolysis. J Neurochem 1992;58:1052-9.
- Kutty RK, Santostasi G, Horng J, Krishna G. MPTP induced ATP depletion and cell death in neuroblastoma×glioma hybrid NG 108-15 cells: protection by glucose and sensitization by tetraphenylborate. Toxicol Appl Pharmacol 1991;107:377-88.
- Cohen G, Kesler N. Monoamine oxidase and mitochondrial respiration. J Neurochem 1999;73:2310-5.
- Chan P, Langston JW, Irwin I, DeLanney LE, Di Monte DA. J Neurochem 1993;61:610-6.